
Why Science Matters or How to get a PhD in Two Weeks
Transcript
- >> Richard Cerione
Good evening.
I'd like to welcome all of you to the Efraim Racker Lectureship in Biology and Medicine.
And especially, I'd like to welcome Ef's daughter, Anne, and her husband, John, who have been here for every one of these lectures over the years.
This lectureship was initiated in 1992 with the intention of bringing to the scientific community, into the general public at large, examples of the major developments in biology and medicine by those individuals who have had extraordinary impacts in those areas.
And in that regard, we've been very fortunate over the years to have had a truly outstanding group of lecturers including Sydney Brenner, David Baltimore, Bob Weinberg, Joan Steitz, Harold Varmus, and of course, tonight's lecturer Tony Pawson.
What we've strived for and I believe what we've succeeded in doing has been to identifying individuals whose contributions in scientific impact truly epitomize the breadth of Ef's research interest in-- the fundamental contributions that Ef's laboratory made, the basic biochemistry into a molecular understanding of human disease.
After a distinguished career at New York University and then at the Public Health Institute at New York, in 1966, Ef came to Ithaca as the Albert Einstein Professor of Biochemistry and as the Chair of the then section of biochemistry here in Cornell.
He was truly an inspiration to the many postdoctoral fellows and graduate students who trained with him and in the numbers of individuals who would leave his lab to go and embark on their own independent research careers.
And I can really tell you from first-hand experience, he was an absolutely terrific mentor and a wonderful friend.
Ef did science as if they were an art form.
He relied every bit as much on intuition and vision as he did on technical knowledge and in literature details.
And that probably comes as no surprise that those who were close to him because they know that one of his true loves was art.
And so throughout his career, he would really combine art and science in an almost seamless fashion and he was absolutely prolific in both of those arenas.
Ef's scientific interest were directed at understanding the fundamental steps of a variety of biochemical pathways including oxidative phosphorylation, neurotransmitter release, the regulation of enzyme catalysis, and the molecular mechanisms underlying the control of cell growth and how the loss of that control can lead to cancer.
And what he really revitalized was always known at that time as "The Warburg Hypothesis" where it was realized that tumor cells showed excessive metabolic activity.
He spent a good deal with his career trying to really understand the links between metabolism and cell growth control.
And this is something that has come full circle now because it becomes a very, very hot area in cancer research.
As those of you who knew Ef, he had a presence that we haven't been able to replace here.
But our hope has been that the spirit of Ef's scientific approach is love for basic research and how fundamental discoveries can be applied to alleviate disease and human suffering.
We'll continue on through this lecture series, and so, we're very pleased tonight to be hosting Tony Pawson.
And now, David Shalloway will formally introduce Tony.
[ Pause ]
- >> David Shalloway
The concept of signal transduction within cells, the process by which a cell converts one kind of signal or stimulus into another, it's so common place now that one might suspect that it goes back to the beginning days of cell biology.
But that is not so.
The phrase only began to appear in research articles after the advent of recombinant DNA which gave us the ability to start twinking individual genes and their proteins and to start looking in molecular detail at the interactions between the biomolecules that cells use to carry signals.
In the early days, which isn't that long ago, as more and more proteins have participated in signal transduction were discovered making any sense of the complexity of interactions became more and more challenging.
So it was extremely important when another concept emerged that could help us to begin to understand the emerging labyrinth of molecular interactions.
This was the idea that signal transduction proteins could do to a significant extent be understood as the assemblages of protein domains.
Each domain being a modular component having a specific type of interaction often non-catalytic that was imported for signaling.
And the start of this understanding was a discovery by Tony Pawson's group in 1986 of what came to be called the Src Homology 2 or SH2 domain, a small protein module that acts as a specific type of binding hook wherever it shows up in the universal proteins.
Since that time, Tony's laboratory had made enormous contributions over a broad front in the area of signal transduction and the mechanisms of cell regulation.
He is currently a distinguished investigator at the Samuel Lunefeld Research Institute at the University of Toronto, and he's one of the 25 most cited authors of the past decade.
You can read in your program some of the numerous awards that he has received in recognition of his scientific contributions and I will repeat them all.
And for one of the most recent was his appointment last year by Queen Elizabeth II in the order of the Companion of Honour.
This is an exclusive honor which is limited to a maximum of 65 members worldwide and recognizes the outstanding achievements in the arts, science, politics, industry, and religion.
So without further ado, I'm delighted to present Tony Pawson who would tell us why science matters or even more importantly, how to get a Ph.D. in two weeks.
- >> Tony Pawson
Thanks David.
[ Applause ] Thanks. Well, it's-- is my mic turned on?
You can hear me okay?
>> Yeah.
>> Great. Okay.
So, thanks.
It's just a terrific honor-- privilege to have a chance to give the Efraim Racker Lectures actually with John Castello to, you know, be so generous with hospitality.
Ef as David Shalloway told you, he was really one of the preeminent biochemist of the last century.
And he was certainly well-recognized in the city where I live, Toronto.
He won, I think, the Gairdner Award in 1980, which is kind of those most prestigious scientific prizes.
So we certainly valued his contribution in the Great White North basically.
I was being mindful of Ef's famous remark, "Don't waste clean thinking on dirty enzymes." Though I must confess that much of my work has involved the notion that clean enzymes are rather sticky and have lots of proteins bound to them so I actually wasted a lot of dirty thinking on dirty enzymes as it were.
So that's been-- this is the first time I'd been to Cornell.
This has been a hugely enjoyable day and I would put more excitement tomorrow.
So that part of my talk will be somewhat philosophical but [inaudible] I am as you're looking at a doctor of philosophy so I guess that's-- I hope that's okay.
And I will also briefly discuss some of the science that I've been interested in over the years.
So you may wonder my title.
You know, first, the question that-- the ideal one needs to question, why science methods may seem entirely superfluous to an audience such as this.
But I have to convince you that it is an important and a relevant issue.
Though I am usually embarrassed to confess that some of my talk will be more like-- that should be re-titled "Why Science Matters to Me?" And you may also think that my subjects try to get a Ph.D.
in two weeks was designed as a shameless ploy to appeal to the students who might automatically had to get a degree quickly and painlessly.
And he would be quite right.
That was my plan.
[Laughter] I will reveal all in due cause but unfortunately, only at the end my lectures.
[Laughter] Let's just wait through the rest.
So my ruminations on the nature of scientific discovery were prompted in part by a comment made by the Canadian novelist, Margaret Atwood, who you may or may not know.
She is really a Canadian icon.
And I think I can fairly call her famous because her name was the answer to a clue in the New York Times crossword puzzle, the clue being something like "novelist in a forest," at wood, Atwood.
Okay. When I call your-- that must be the peak of fame as one can get through.
So in an article published a couple years ago in Canada's main newspaper, the Globe and Mail, she [inaudible] as shown on this slide, "Arts are at the heart of the man.
Science is about knowledge.
It is only a tool." So this is a sentiment that I disagree with, in fact so and hence my introductory run.
So you might dismiss this as a misguided view of one person kind of well-educated of culture.
Perhaps, it was made for dramatic effect.
But I would argue that it's an insidious opinion that is widespread even among people who ought to know better, including many of our politicians.
I say young politicians in Canada.
Now, that's American politicians are more and more.
[laughter] And I think it represents a troubling distortion of the scientific ethos.
So I would assert to the county that inquiry and discovery, in other words, science really lies at the root, lie at the root of what it means to be human.
We, human beings, I think are driven to learn and explore.
We want to know where we came from, we want to know how living creatures work, and we want to know how the universe that they inhabit is constructed.
To blur from Alexander Pope that to him somewhat out of context presumed not to go to scan the proper study of mankind is man.
So in my experience contrary to what Margaret Atwood would argue, one of the greatest joys in life is to make a new scientific finding however small.
And certainly, I have a sense that you are looking at something about the world for the first time.
Then I fantasize that this evokes the same sensation as the discovery of new continents, for example, by early explorers.
So this sublime feeling for wanting a better world is to my mind what science is really about.
And I can best make my point by describing one of my first students in the 1980s when I was at the University of British Columbia, our fellow called Ivan Sadowski, who is now a professor back at UBC.
So Ivan grew up on a farm in Saskatchewan.
And even his best friends would say that superficially, Ivan has a somewhat down personality, let's say.
But he is a technical genius.
He could get any piece of equipment in the lab to work.
And when I asked him how-- where he came by this talent, he replied in somewhat rough way.
"Well if you grown up fixing tractors of 20 degrees below zero, you will be able to get things to work too." And that was [laughter].
So when he was doing his Ph.D., Ivan discovered this element characteristic of proteins involved in cellular communication that David alluded to, the SH2 domain that I will briefly discuss later in my talk.
So at the time he was undertaking this work, we had to go to another building to develop the x-ray films that would tell us the-- reveal the results of our experiments.
So Ivan would give up for this x-ray films and I'd wait on [inaudible] as well, develop the films and then trudge back.
So he reappeared one day in 1984 with a film that-- a developed film that provided the first hints of the SH2 domain, and you can imagine that I was somewhat thrilled.
I jumped up and down.
Ruth opened a champagne.
And I was a bit surprised that Ivan appeared completely unaffected.
So I asked him, wasn't he a least bit excited?
To which he replied, "Well, I did turn a cartwheel on the way back." [Laughter] And so, to my mind, turning cartwheels epitomizes why we do science.
I mean it's that sense of thrill and excitement that you've really discovered something.
And so I think that is one reason that science matters.
It isn't a tool.
Rather, in my view, it manifests the vibrancy and creativity and wonder, if you will, of the human spirit.
It's clearly as a music or painting or literature.
So I had actually, you know, appreciated the extent of Ef Racker's sort of wonderful artistic talent.
But he clearly didn't see any distinction between scientific and artistic endeavors.
Lewis Thomas, who was-- you may or may not know was one of the great American clinician scientists of the last century, captured this emotion very well, I think, in describing the extraordinary fact that complex organism such as ourselves arrives from a single cell.
You may not remember that you were once a single cell, but it is nonetheless-- true nonetheless.
So he wrote about-- oh, sorry, gosh.
We had-- so, you're awake now?
[laughter] Yeah?
So he wrote about this process of scientific-- of embryonic development.
No one has the ghost of an idea how this happens.
If anyone figures it out in my lifetime, I will hire a skywriting airplane, maybe a whole fleet of them to put one exclamation point after another across the whole sky until all my money runs out, which is sort of the most wonderful description I have ever heard.
This would have thrilled the unknown and the excitement about the possibility that it might be sorted out.
In his lifetime, Lewis Thomas never had the opportunity to so enrich some lucky skywriting pilots.
But as you may know, the secrets of embryonic development are now starting to be revealed.
But it-- to my mind, it's something that really still literally bugles the mind.
The notion that one cell can have the self-organizing principles to create a multicellular animal.
So here, for example, is a mouse embryo at about embryonic day three and a half.
So this is where just before its starting making what developmental biologist call a cell fate decision, where some of the embryo is going to go on to become of the embryo proper and other cells are going to become the extra-embryonic tissue.
And so you see here the expression pattern of genes or rather their products that are going to then control the cell fate decision.
I find this one of the most fascinating things that one can think about.
And I think it's a phenomenon that will seem even more remarkable when we fully understand it.
So scientists, this is one of my points, are passionate about what they do.
They dream and they imagine.
And I said before, I think the zest for discovery and exploration is an innate human trait.
And in fact, I think we wither if that-- the ability to make those sorts of explorations is denied.
So continuing my analogy between science and exploration, the spirit of discovery, I think, is embodied by the following gentleman, an explorer, not a scientist.
Ernest Shackleton, who you may or may not know, who in 1913, placed the following advertisement in the London Times trying to recruit people to accompany him to the Antarctic Continent.
So when I show this, you may find this rather reminiscent to how it feels to be in graduate school.
So he said, "Men wanted for hazardous journey.
Small wages, bitter cold, long months of complete darkness, constant danger, safe return doubtful, honor and recognition in case of success." [laughter] You know, we've all been there.
And the thing I particularly like about, it's I think an old-fashioned use of the word "honor." It means-- in this context, I think that it's sort of giving everything that one has as fulfilling of-- a fulfilling of one's potential.
So Shackleton, as you can imagine, was immediately inundated with applicants, which is the true.
But it's only fair to point out that the explorer-- that the expedition was a complete disaster.
So you see here the endurance, his boat trapped in the ice.
So I think that's definitely an experiment that went wrong.
But then true heroic efforts including one of the longest and most dangerous voyages that ever made in a small boat, he brought his entire crew home safely.
So my point, in case you've forgotten, the point I'm making is that science is a full of exploration, I think, that satisfies something important that we as human beings need.
So this brings me back to Margaret Atwood's contention that science is only a tool.
So, why is this a dangerous concept?
I mean why can-- what Margaret Atwood might think?
And I believe that this kind of thinking can encourage funding agencies, governments to be too rigorous in directing and channeling the efforts of scientist to the detriment, I think, of really innovative research.
So in Canada, I mean this may not be true in the US but, you know, the virtues of translation either to the clinic or to the industry extolled as paramount and basic research is increasingly considered a luxury.
We, in Canada to a significant extent, judged by the perceived socioeconomic benefits of our research, our training of highly qualified personnel, which I'd like to abbreviate as HQP, and/or submitting off detailed quarterly reports.
This, I think, can be counterproductive in fostering new unorthodox or theoretical ideas.
So I don't in anyway mean to decry the importance of finding practical applications for fundamental research as I hope to make it clear shortly.
But it is worth pointing out that the early members of Britain's Royal Society, the founders really of the experimental and fact-based age of enlightenment encouraged and here, I quote from John Gribbin's history of the Society's early years, "The speculative investigation of the world by people interested in knowledge for its own sake, not for its practical utility." So this leads to a second realm in which science is more than simply a tool.
By breaking up our preconceived ideas, scientific insight can completely change the way we think about ourselves.
For example, we biologists must view the world through the lens of evolution but it goes deeper than that.
Darwin's discoveries were so profound that they altered forever a sense of relationship to other living organisms.
So these kinds of advances, these kinds of changes can initially be uncomfortable.
And if they are really important, that discomfort can linger as we see with the creationist and the intelligent designer.
So-- and one can have perhaps some sneaking idea of the issue which Sydney Brenner, I think, a former Racker lecturer has commented on.
Sydney said that the man on the street feels that the process of evolution driven by random mutation can't really work because he knows that if you take a black and white TV and you hit it with a hammer, you very rarely get a colored TV at the end.
You know, but ultimately, these new paradigms come to permeate the intellectual air we breathe.
And I think if their birth is stifle by too much direction and too much political oversight, too much attention to the completion, predetermined milestones, society will inevitably stagnate.
So by definition, obviously, it's hard to predict the unknown.
So to return to the early fellows of the Royal Society of London, they, in fact, viewed discovery and its application as two sides of the same coin as is evident from one of Robert Hooke's famous to-do-lists of which I have a slide.
So, you know, Hooke sort of doodled about what he might discover and, you know, what it might be useful for improving, you know, engines for trade, engines for carriage.
He sort of left out what the heavens might be useful.
[laughter] And you know, you have the sense that he even really distinguish between basic or applied or-- so-- and I think this increasing the case in current biomedical research where results obtained from patients in the clinic inform the underlying basic science and vice versa.
So I'll discuss in the moment the research that has led to the use of new anticancer drugs.
But it's important to remember that the clinical data has not only it inspired further basic experimentation but they have told their own scientific story.
For example, as we try to understand how resistance to anticancer drugs is generated, these kinds of things tell us a lot about the basic science.
So it's a two-way street.
But I would assert that this-- it's not easy to manage this process of translation on a large scale especially in the biological sciences, the article's spectacular exceptions such as the sequencing of the human genome.
But I think that for the immediate future, this kind of thing will be the exception rather than the rule.
So in part, this sort of large scale targeted approach to biology usually doesn't work I think because we are so ignorant.
And so on this point, it's worth going to back to the clinician scientist Lewis Thomas talking about the discovery of antibiotics.
So he wrote in 1980 about the discovery of antibiotics.
I was a medical student at the time of sulfanilamide and penicillin.
And I remember the earliest flat rejection of such things.
We have given up on therapy a century earlier by which he means that in the 19th century and early part of the 20th century, physicians had sort of figured out that if they actually didn't prescribe much of anything for the patients, many of their patients got better any way and often did better than if they did prescribe them.
But they become brilliant diagnosticians so they could tell you what you have and what was the percentage chance of what would happen to you.
But the idea that as he goes on to say, that the realization that disease could be turned around by treatment provided that one knew enough about the underlying mechanism was a totally new idea just 40 years ago.
So he was writing this in 1980 which was some us seems not so long ago.
And so easily within living memory, the idea that a physician could prescribe a mechanism-based drug that would cure a patient was considered outrageous.
And as he said that there was a flat rejection of such things.
So I think we now suffer from the opposing view that it ought to be able to-- we're not to be able to cure everything.
And you know, one often finds talking to one's friends, you know, well why haven't you guys figured this out yet?
And the answer of course is that these are early days that we've made great strides in medicine.
But we are so relatively inept in dealing with many diseases especially diseases like cancer and those that affect the nervous system.
So for example, we can tell a patient that he has a mutation that means he's going to develop a neurological disorder such as Huntington's disease, and we can estimate from the nature of mutation how severe the effects will be.
But we cannot yet effectively treat the disease once it appears because we simply don't know enough.
We can give a kinase inhibitor, a drug to a lung cancer patient with a mutation in the gene for epidermal growth factor receptor.
And the patient will respond for awhile but almost always will relapse.
So to obtain more breakthroughs in these fields, we need to fund bright and passionate people with fresh ideas.
And in the latter case, the answers may lie in better understanding intercellular networks or compensatory oncogenic events, or stem cells, or the ability of tumors to attract the blood supply, or the metabolism of tumor cells as Ef Racker was so devoted to or all of the above, and certainly, something that we haven't even thought of as yet.
So a third reason to my mind that science matters is, if you will, is that it does indeed lead to new practical applications but not in the sense of simply inventing a tool.
New concepts have to be discovered.
New ways of thinking have to be introduced before the tools have a chance of working.
The other reason that I don't like the tool analogy is that it implies that a problem is obvious, simply awaiting a practical solution, but this often not the case.
So the relationship of smoking to lung cancer, for example, which we now take completely for granted, was only recognized after pioneering epidemiological analysis by Richard Doll and others.
So science identified the fact that there was a problem in the first place which we are now trying to solve by the development of drugs such as kinase inhibitors.
And this was really brought home to me very forcefully passing through the lobby of the hospital where I work recently.
So a raid in the lobby of paintings of the chairman's of the-- chairman of the hospital board in all their glory.
So, two of the chairman of board from the 1970s had their portraits painting holding cigarettes.
So, you know, it-- you know, and that's something today obviously that would cause a righteous outcry.
So this epidemiological science didn't simply, you know, reveal something that everyone sort of suspected in the first place.
It really turned all our ideas upside down since evidently these worthy is being painted for a hospital, thought that holding a cigarette in their hands was a badge of honor in a sense.
So, you know, the same is true for issues like climate change in the sense of science alerting us to a new problem.
It's not that everyone realizes that initially there's a problem which we must now invent tools.
Science reveals the issue.
So the notion that the atmosphere traps heat, the so-called greenhouse gas effect, was mooted in the first part of the 19th century.
And in 1896, as you may know, Svante Arrhenius, that might actually the slide.
Yeah. He deserves his own slide.
Was the first to propose that man made increases in CO2 could lead to warming of the planet in a paper that prophetically entitled "On the influence of carbonic acid in the air upon the temperature in the ground." So his paper did not cause immediate alarm because he anticipated the global warming would take around 3,000 years, which one source I located cryptically called an underestimate.
So his prediction that of the extent of warming that would be induced by doubling CO2 concentration in the atmosphere is inline with current models but he didn't foresee the rapidity with which CO2 levels would increase.
And actually, he thought it might be a good thing because he thought a bit of warming for Sweden would not be a bad idea.
So the ice cold records as you probably know showed at the start of the industrial revolution in about 1780, CO2 levels in the atmosphere around 280 parts per million and had likely been at this level for a millennia.
The CO2 level in the atmosphere rose to 330 parts per million by the 1790s to 360 part per million by the-- excuse me, in 1970s, 360 parts per million by the 1980s.
And today, it's around 380 parts per million.
So here we need science not as a tool but as a means to open our eyes to what's really going on in the world and to predict its consequences and to reliably inform us and politicians as to solutions.
So in the case of climate change, of course, this is a difficult issue because we're dealing with a long-- nonlinear system but it's important stuff, and again, discoveries have to be made.
So science has and my sort of last comment along these lines is that science has another feature that I think will be increasingly important in the modern world.
Science is, you know, and I can-- you know, I started my career in the UK then I worked in the US.
Now I work in Canada so I've seen a little bit of international science and science is clearly a global endeavor.
And scientists around the world share the same objective view of things.
So I think this is potentially a remarkable source of mutual understanding in an increasingly fractured land-- fractured world rather.
So the typical lab in North America has scientists from all corners of the earth working collaboratively.
And I'm hard put to think another vocation or profession with this kind of sort of global reach if you will.
So in my lab, I'd hear French, German, Hebrew, Danish, Australian, that's right.
That was a joke, spoken almost as much as English and my senior technician is Nigerian or Nigerian-Irish.
So I think this sort of supranational structure of science is really something we need to encourage.
And curiously, certainly in biological research, funding is almost all at a national level in finding resources for international collaborations, unless they are mega projects, are very hard to come by.
There are few exceptions like the Human Frontier Science Program.
But it sort of remarkable when I talk with scientists in the US or in the UK and/or Australia, where it maybe and we said "Well, how could we get funding?" They said, you know, it's hard to come by.
So we're a global society but-- and I think, you know, there's work to do in really sort of realizing the power of that.
So if you would allow me to indulge myself for a few minutes, I'd like to talk just a little bit about some of the science in my field as cellular communication and how it might relate to at least a few of the themes that I have introduced.
So, you know, obviously for 99.9 percent of you, this will be an unnecessary slide.
But just let me remind you that the genetic material in your cells, the genes in your cells is decoded into RNAs and into proteins.
And I show here the representation of the three-dimensional structure of a protein that we've been interest in.
And what you can sort of see at a glance is that proteins are much more complicated beast than is DNAs.
Proteins and RNA of course that do the work in the cell that organize the cell.
So thousands of proteins then associate, interconnect to make a giant sort of network, if you will, that forms the cell.
There's an experiment actually.
I'm going off message here.
But there's an experiment that I thought about doing for many years which would of course lead to a paper in the cell nature, one of these learned journal, which derives from the early source of experiments that David was alluded to where we were looking at the interactions of proteins one with another.
And skeptics would say to me, "Oh, you know, you guys, you just think everything interacts with everything else." So the experiment we have been following, one would take the suspension of cells and one would cool the cells down, let's say with an antibody to some extracellular part of the protein.
And then you would separate the cells, the resulting proteins on a 2-D gel let's say and analyzing by mass spectrometry and make the amazing discovery that everything in the cell is indeed connected with everything else.
So how-- you know, how does this work?
And an important observation which again, I was sort of brought up to think of cells as being sort of, you know, bags with lots of water inside and a few organelles swimming around.
But of course, that's not the case.
So here you see the side of skeleton or part of the side of skeleton, the cell we see is something incredibly density packed.
So the inside of the cell is a very crowded place made up of proteins, RNA, lipids, phospholipids and many other biomolecule.
So the question we've been interested in over a number of years is how are cells organized and how do they communicate with one another, and then can one use that information to learn something useful.
So as you know, cells in the body communicate through a language involving the expression by one cell of let's say a hormone, a protein encoded by a gene.
And that the only reason that that hormone has an effect on its target cell is that the target cell expresses a receptor which I've shown diagrammatically here or also protein which crosses the other membrane of the cells that's got a bit that interacts with the seedling molecule on the outside and then a bit within the cell that will signal for a response.
So the only one reason that that cell response is that it has a receptor.
There's no intrinsic seedling information in the hormone in the absence of a receptor on the cell surface.
So then I've been interested in the question once these receptors are activated, how do they communicate with the core machinery within the cell to alter things like cell shape, or the expression of genes, or the regulation of cellular metabolism, or survival or cell growth?
And a simple way to do that and, in fact an approach that the cell uses, is to evoke proteins, sort of coupling proteins if you will, or adapters that have this kind of modular design that David alluded to that composed of elements, what we called domains, that recognize on the one hand some aspect of the activated receptor.
So in this case, I put a P here to indicate that this receptor has become phosphorylated by the addition of phosphate groups once it's activated.
And then one or more additional domains that selectively recruit some aspect of the-- sort of core cellular machinery.
So this is a-- in principle or sort of rather simple way in which information might be communicated from the outside of the cell to the inside.
And in fact, can allow also to responses to changes within the cell, for example, DNA damage sort of works in rather the same way to signal to the cell cycle apparatus.
So just as a, you know, to sort of take you one step further and I apologize for dwelling of this, but-- so here we've got a couple of transmembrane receptor molecules.
Here's the outside of the cell and the inside.
And here's a hormone, in this case, a hormone platelet-derived growth factor and so the hormone is going to bind to these two receptors which are then clustered.
And the consequence of this clustering is that it allows two adjacent receptor change which are indulge with the catalytic activity which allows them to phosphorylate the amino acid tyrosine, one of the amino acids found in this protein chain.
So as a consequence of that proximity, they then cross-phosphorylate one another and that stimulates their intrinsic catalytic activity.
Such that additional tyrosine residues become-- tyrosine amino acids become phosphorylated and that leads to the engagement of downstream targets through their ability to recruit proteins like these adaptors.
And the way that they do that is they ever told you is by this site, once it's modified, so this is a dynamic process requiring the addition of phosphate to the tyrosine creating a docking site, a binding site for the SH2-- so called SH2 domain of an adaptor that can then communicate with additional domains with downstream target.
So the SH2 domain directly recognizes this phosphotyrosine containing site.
So if one sort of takes that bit of the cartoon and looks at it from a sort of structural point of view, here's a structure of a particular SH2 domain that John Kuriyan solved some years ago, and it has sort of run the-- to my mind sort of beautiful organization.
So he is the SH2 domain itself and it's bound as you may be able to tell to a phosphotyrosine-containing peptide.
So imagine that was-- the peptide is this fragment of deactivated receptor.
And you can see perhaps it is divided into two-- sort of two binding pockets, one of which binds to the phosphotyrosine and requires that that tyrosine have a phosphate group stuck on it and that's why this interaction is a dynamically regulated one.
And then a more variable surface that recognizes the amino acids that follow the phosphotyrosine and this confers an element of specificity of cell activity in this interaction.
So this is-- was a sort of prototypic example but we now understand that about 70 percent of human proteins have this kind of modular organization and many of those modules are domains that allow the recognition of other proteins, or small molecules, or phospholipids, or nucleic acids.
And so this is a rather general sort of organizing principle, if you will, that allows for dynamic cellular behavior.
So, you know, one of the reasons for being interested in this is that this kind of signaling process can be a critical step in the development of diseases like cancer.
So in a normal cell, in the absence of its appropriate growth factor or hormone, our receptor is inactive.
But then as you may know, mutations that affect the receptor in a way that sets it into an active state even when there's no hormone around leads to it's recruitment of SH2-containing proteins, for example, and the induction of a downstream response in the absence of a signal.
So I think of the cell as being sort of, you know, deranged in a sense it's hearing voices.
It thinks it's being told to grow when in fact there's no signal out there.
And so this kind of paradigm has then led to the development of targeted therapies, for example, the receptor HER2 or otherwise called ErbB-2 or Neu is activated because it's very highly expressed in a significant fraction of breast cancers that tend to have a poor prognosis.
And this antibody therapy, Herceptin, is directed of that cancer-causing protein.
The one other story that has-- that I'll briefly allude to and that has sort of underscored this paradigm involves the cancer chronic myelogenous leukemia, which results from the breakage of chromosomes and their realignment so that chromosomes 9 and chromosomes 22 become joined to one another in an aberrant way.
And as a consequence of that aberrant joining of the chromosomes 9 and 22, a gene and its product called BCR becomes joined to a tyrosine kinase related to those growth factor receptors that I was alluding to.
Though in this case, an intercellular protein that you notice has not only a catalytic tyrosine kinase domain but also an SH2 and its routed on SH3 domain.
So in this chronic myelogenous leukemia cells, there this aberrant abnormal BCR-ABL protein expressed which is never found in a normal cell.
So this region, this BCR region at the start of the protein results in the BCR-ABL protein forming an aggravate, if you will, and that results in the activation of the kinase domain and then there is a series of binding events of the sort that I was alluding to that are important for the transmission of a downstream signal and that leads to leukemia.
So, one of the real advances in this field was the discovery by Novartis of a drug Gleevec that binds to and inhibits the activity of the catalytic kinase domain of BCR-ABL.
And that has really a striking therapeutic.
And in some cases, you know, also the patients are only seen seven years out or so, but once tempted to say, a curative effect.
So here's the structure of that complex and the green ribbon is the ABL kinase domain and here's the drug Gleevec, which you can see is sort of rather like an apple that's been sort of stuffed in the [inaudible] of the kinase, the constant chew on its substrates.
So, the other-- another cancer that Gleevec has been at least partially used for in treating is a tumor called a gastrointestinal stromal tumors are rather rare, very malignant tumor, which can arise as a result of mutations in a receptor of the sort that I was alluding to with the unusual name of KIT.
So mutations in the gene and that's alterations in the protein of this receptor, the KIT receptor, causative for gastrointestinal stromal tumors.
So-- and KIT then is inhibited by this drug Gleevec.
So here's a patient, an OB patient before the administration of Gleevec, and you can see the tumor is being imaged and then fairly shortly after Gleevec has started, the tumors have shown a remarkable remission which you'll also see here on this image.
So, you know, this was really I think a striking discovery which took many, many years of basic science and led to the identification of a drug that does have therapeutic effects.
I borrowed at least conceptually a slide from Tony Hunter to make the point that I was arguing earlier that it's hard to manage these things.
So chronic myelogenous leukemia was first described in 1845, that sort of breakage of chromosomes was described by Janet Rowley and others in 1960.
And then there were a series of important sort of basic science observations that ultimately culminated in the isolation of this compound Gleevec and then its testing in patients.
So it-- you know, it's hard to hurry these things, which is part of my argument.
So I'd rattled on to long so let me just-- I think I'll stop the-- my discussion of science then.
And so those of you who wanted to know how I got a Ph.D.
in two weeks, okay, I'll tell you.
So I did my undergraduate work at Cambridge University and I was mentored by a fellow called Tim Hunt, who was honored along with Paul Nurse, I guess, with the Racker lecture.
I've just recently put his work on the cell cycle.
So Tim when I sort of finished my undergraduate career recommended he's going to Ph.D.
with a fellow called Alan Smith who worked at the curiously named Imperial Cancer Research Fund in London, England.
So I applied and was accepted to do a Ph.D. in Alan's lab.
And what I haven't really realized is that the-- I see a requisite was called at the time was composed almost exclusively of principal investigators and post-docs and I was one of the very first graduate students they took on.
But, you know, that was okay.
So I did some work.
I published a few papers and after I've been three years.
So the time in the UK, they gave me three years to write a thesis and get in.
And so after I've been there, let's say two years and ten months, well, so Alan said "You better write your thesis" so I wrote my thesis and then threw it back to him and he said, "All right, this is tricky." And he said, "Well, so which university registered that?" You know the phrase, "his bowels turned to water," I mean, that-- yeah.
I said, "You didn't tell me about that Al." [laughter] And, you know, so he said, "Okay.
Well, don't worry.
I'll, you know, I'll call around." So he called a friend of his Kings College, London and they said "No problem." So I went around, I spent the whole morning back registering for three weeks and then two year-- two weeks later I took my exam and got my Ph.D.
So [laughter] formally I got my Ph.D. in a couple of weeks.
So let me just sort of finish then, you know, so that I start out.
Though I should say, it's a little disconcerting.
The Imperial Cancer Research Fund is now called Cancer Research UK, so it doesn't formally exist anymore.
So I got my Ph.D.
from an institution that I only visited once from the university.
I on visited once from an institution that doesn't exist anymore but I do have a Ph.D. certificate.
So, one consequence of being Imperial was that the queen would visit on occasion.
In one such event, she was introduced to one of my other mentors, Steven Martin, and she asked Steve what he worked on.
And he replied, "I work on viruses that cause chillers in chickens, Ma'am," to which she responded, "Oh, how interesting." [laughter] So one can forgive her majesty perhaps for her apparent skepticism.
So how could she have foretold that such blue sky research into an odds chicken virus would have been crucial on the one hand for a subsequent understanding of another retrovirus, the human immunodeficiency virus, and that's for the development of drugs that treat AIDS.
And on the other hand, the discovery of cancer genes, a new targeted therapies for human malignancy, the sort that I have described.
So that I would submit to you is why science matters because of the unpredictable truths that it reveals about the world.
So I really don't believe in this duality of arts and sciences or discovery and application.
I think they are old threads of the same fabric of human inventiveness.
And if I could finish by putting the Nobel laureate in Chemistry, John Polanyi, who works in Toronto, he published recently an article linking the pursuits of music and science in a rather portentous fashion.
But I'd rather like he says, "Every scientific proposition and every musical composition is aimed at eternity.
What is true, we believe, will outlive the sun." It's terrific.
[laughter] So anyway, I apologized for rattling on some and I'm really-- thank you for the honor again to give this lecture.
[ Applause ]
About the speaker
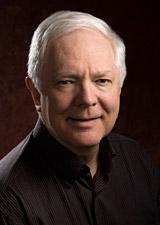
Anthony Pawson
Distinguished Investigator, Samuel Lunenfeld Research Institute of Mount Sinai Hospital & Department of Medical Genetics and Microbiology at the University of Toronto
Tony Pawson has pursued the mechanisms by which cells in the body communicate, and the aberrations in the process that result in diseases such as cancer. In particular, he has focused on the means by which growth factor receptors at the cell surface are linked to their intracellular targets. This led to the identification of the Src homology 2 (SH2) domain, the prototypic protein interaction module that exerts its effects through the selective recognition of phosphotyrosine sites on activated receptors. It is now apparent that modular protein-protein interactions control many aspects of intracellular signaling pathways, and provide a general architecture for organizing cell regulatory systems.
Tony Pawson obtained his Ph.D. at the Imperial Cancer Research Fund (now Cancer Research UK), working on retroviral gene expression. He undertook postdoctoral work at the University of California at Berkeley, where he identified a variety of retroviral oncogene products, and provided early evidence for the role of tyrosine phosphorylation in malignant transformation. He moved to the University of British Columbia, Vancouver as a Assistant Professor in 1981, and then to the Samuel Lunenfeld Research Institute of Mt. Sinai Hospital, University of Toronto in 1985, where he was Director of Research (2000-2005). Over the last 20 years he has explored the mechanisms through which signaling networks within the cell are constructed, building on his discovery of the SH2 domain, and the ways in which such networks are utilized to develop functional tissues, as well as the defects in molecular interactions that promote human disease.
Dr. Pawson is a University Professor of the University of Toronto and a Distinguished Scientist of the Canadian Institutes for Health Research. He has received many awards, including the Gairdner Foundation International Award, the AACR/Pezcoller International Award for Cancer Research, the Heineken Prize for Biochemistry and Biophysics, the Louisa Gross Horwitz Prize, the Wolf Prize in Medicine, and the Royal Medal from the Royal Society of London. He is a Fellow of the Royal Societies of London and Canada, a Foreign Associate of the National Academy of Sciences (US), and an Associate Member of EMBO. He was recently appointed by Queen Elizabeth II to the Order of Companions of Honour.