Efraim Racker

The Making of a Scientist: An Unlikely Journey
Transcript
- >> Rick Cerione
Well. Good evening and I'd like to welcome all of you to these years Efraim Racker Lecture in Biology and Medicine.
This was a lectureship that started in 1992, really with the intent of bringing both to the scientific community into the general public at large, some of the examples of major developments in biology, and medicine by those individuals who were responsible for these accomplishments, and we've been extraordinarily lucky in terms of having-- had a truly an outstanding list of lecturers starting with the very first lecturer Jim Watson, who turns out to had been the PhD mentor of tonight's lecturer, and others including Sydney Brenner, David Baltimore, Harold Varmus, Paul Nurse, all of which were Nobel laureates as is tonight's lecturer.
What we try to do is to identify individuals whose contributions in the areas of really biology and medicine epitomized as research interest, and really the way he thought about science.
After a truly distinguished career at the New York University and then at the Public Health Institute of New York, Ef came to Ithaca in 1966 as the Albert Einstein, a Professor of Biochemistry, and as the Chair of the then section of biochemistry.
Ef researched centered in the area of really the biochemistry of cellular metabolism and really what happens to cells and really how disease ensues when this metabolism goes awry.
And he was especially interested in altered metabolism as if would pertain to cancer which often referred to as the Warburg effect, really the changes in glycolysis that are characteristics of cancer cells.
And this is an area that's getting a tremendous amount of renewed interest now and to the extent really where really makes me believe that Ef is giving a smile about all this somewhere.
Now he was clearly a brilliant researcher.
He was a prolific painter.
He was a personality.
He had a persona like none I've ever met before or probably ever will.
But where our hope really has been is that his spirit, the way he did science, the way he thinks about science, the way his lab really operated, well, sort of live through this seminar series.
Again, by individuals who do science along those same lines.
And so we're especially privileged, we're very pleased to have Mario Capecchi here tonight who will now be introduced by Volker Vogt.
[ Pause ]
- >> Volker Vogt
So it's a real privilege and an honor for me to introduce Mario Capecchi to you here, as this year's Racker lecture.
He's considered as many of you know the father or at least a father of the-- what's become known as the targeted gene disruption in the mouse or just gene target for short.
This technology underlies much of our recently acquired knowledge and the role the genes play in diseases and the general understanding of genes function in mice and people.
He's had an absolutely profound effect on-- from influence of modern biology.
So Mario has won many awards over the years, and you could read about some of them in the brochure and that was-- this was-- could-- have culminated in a Noble Prize in 2007 and this year [inaudible] two others.
So, Mario Capecchi, just a little bit of background he was an ungrad at Antioch College and after he stay at MIT, he became a graduate student at Harvard and he finished his PhD there in 1967.
Already he's a grad student, Mario was a pioneer.
So he worked in the area that one could say that those of us who knows some of his fields is something of an art event.
So this is the [inaudible] old translation which means synthesis of proteins and crude bacterial extracts.
So this crude systems lead to the eventful understanding of how ribosomes initiate, translation, how he terminate translation really fundamental-- you know, fundamental kinds of knowledge, and has Mario Capecchi's papers with some of his coauthors are classics now, long ago.
For the few years that Mario and I overlapped actually in that school, in same lab, I remember that his research struck me as being-- is a requirement, special care-- carefulness about detail-- attention to detail one can almost say the fastidiousness and I think these are qualities that have always characterized the world's best scientist in our age and the past ages.
So anyway, going back to the history, a little ebit after seven years, I was-- so he stayed seven years at the Harvard post graduation, first as a prestigious junior fellow, and then as a faculty member then he moved to Utah in 1973.
And since 1988, he's been a Howard Hughes professor, which most of you or many of you know is such a great honor.
So after moving to Utah, Mario's lab became interested in the potential utility of introducing DNA into the mammalian cells.
First by microinjection using a tiny needle, a really tiny needle, sticking a needle into nucleus of cells without hurting them and some of these techniques they pioneered.
They were pioneers.
And then later using other methods something which is now usually called-- it is called transfection.
Again, in this lab's works was revolutionary, and most importantly, it showed in the-- that-- this is in the early '80s now, that the-- that somatic cells was cells growing and culture, mammalian cells growing, laboratory the way so many people do, have the machinery to [inaudible]-- homologous recombination.
So that means that joining of DNA molecules together covalently at-- when they have identical sequences.
Then they later demonstrated the extent of this to showing that this process, homologous recombination could occur between DNA that's introduced like microinjection, and chromosomes, and the same sequence on chromosome, and that was revolutionary.
So this lab's discovery led to Capecchi's vision that one might experimentally, you know, just a vision at the time, might experimentally be able to insert-- introduce DNA molecules into chromosome at a specific site, some sequence.
So such site specific insertion seemed a farfetched idea to many people, doing some [inaudible] agencies.
But Mario pursuit it with persistence and ingenuity, he and his coworkers, and again, these are qualities that I think sort of characterize greatest scientist in any age, eventually ever rewarded with success, and they were able to show that [inaudible] introduce DNA could-- could, at a low rate, maybe one in a thousand cells could be integrated specifically, inserted into a chromosome.
So to finally give birth to the present day technologists-- it's now used at which is widely describing every cell [inaudible] in the textbook.
The Capecchi lab used the knowledge here acquired earlier from not to faded this introduced DNA, or transfective DNA to design a clever, double selection technique using drug resistance markers, that would-- could be used to select out, to grab that embryonic stem cells that they work with it later to find those that have specific integration and then they could be used to reconstitute tissues in the mouse that's what's done today.
So today, thousands of genes individually, thousands of genes, not together, have been knocked out, modified in the mouse and those all in consequence success [inaudible] greatest and as you will certainly appreciate most the conclusions can be extrapolated pretty directly to human biology and human disease.
So just to finish this off, I just want to say that I mentioned care and attention to detail, and tenacity, experimental cleverness, I guess you can call it, vision, and some other characteristics of Mario Capecchi's research contributions over this many years.
And I just want to mention two more to finish off, and that's humility and generosity.
So, you know, these are human properties, and they can be expressed in many different ways as we all know, different contexts.
But for scientist, they include that generous acknowledgment of-- sorry, both competitors, because science is very competitive, at least, at the forefront.
Competitors where other people have done who were pursuing the same aims, and also the previous workers, whore years or maybe generation for [inaudible] we assign a stem.
So all of these characteristics including especially the last two I think Mario Capecchi deserves real recognition.
He's truly been a giant in his field.
So let's welcome him to Cornell for this 18th Racker lecture.
[ Applause ]
- >> Mario Capecchi
So, first of all, its great pleasure to be here, and be able to share our work with you, it's actually a privilege and an honor to be authoring Professor Racker, and I think he's a giant among biochemists.
I mean, imagine in 1950s, 1960s, he was working with an enormously complicated machine, not only working with that machine, but that machine required membrane in order to function.
And was able to then extrapolate how this machine was making ATP which was as all of you know, is the currency, the energy currency of living organisms.
So-- And I think the one thing that I want to bring out, and I'll bring it out later also in the lecture is that, you know, the old saying that we stand upon the shoulders of other giants, and I think Racker is one them.
I remember, you know, being as a graduate student, and whenever a paper come out by Racker, you know, I will read it very, very carefully, because I knew I would be quizzed on it.
[laughter] It was monumental at the time.
And so it's a great privilege to be following in his footsteps.
So, what I'm going to do today is give you essentially three stories.
They're going to be very general.
The first is simply a description of what we do as a scientist.
And then, I'll go into thing that's a little bit more embarrassing for scientist, scientist love to talk about science.
But they-- I tell my students the reason they're going into science is because they cannot communicate with other human beings.
[laughter] They're comfortable to the bench.
And so-- And they're particularly uncomfortable when they have to talk about themselves.
And so if he was here [inaudible], that's why.
And then finally I'll take you just a quick trip along to stop.
So that's sort of the outline.
So, what do I do as a scientist?
I think all of you are aware, I mean everyone of our cells we have an extremely complicated instruction manual.
It's composed of three billion letters, it's an alphabet of four letters, rather than 26, and the order of those letters is the text.
And you're also all aware that the text is written, is held together essentially by this molecule, DNA, which is actually are marvelous structure.
So the backbone is holding all the letters, A, C, T, G, and the steps essentially are the letters.
And this structure was worked out by these two characters, age 25, age-- early '40s, Watson and Crick.
And as you've heard, he's the mentor of several of us in this audience.
Credit should also be given to this person 'cause it was her data, Rosalind Franklin who actually provided the x-ray photographic data upon which the structure was obvious then a double helix allows essentially. Jim Watson had a photographic mind.
He saw that picture, he could then recapitulate that picture in great detail when he was talking to Crick, the [inaudible].
But I think had she lived, she would have certainly would have shared the prize.
Now, what makes DNA interesting and it's actually basis on everything we do, technically from then on is this property, that is the-- if there's a thymine-- these are the two strands, the backbones, and then these are the letters, the basis.
So if there's thymine on one strand, then the opposite strand will always have an adenine at that position, and if it's a cytosine then there'll always be a guanine, okay.
So, it's this rules essentially which maintains essentially the equidistance of the two backbones of the DNA Helix, which then are accountable essentially for the properties of DNA.
And that was meeting [inaudible] once they simply saw the structure, and had created it.
And for example, it tells right away how DNA is replicated, because all you have to do and why it's the ideal essential archival molecule.
You separates the strands, and then you can simply use them as a template for making the opposite strand.
And so there-- and that allows essentially-- two daughter cells coming from the original cell to have the exact set of information.
And the process is enormously faithful, it makes-- I've mentioned that there are 3 billion base pairs, roughly one-- that basically are changes made doing replication per billion based pairs.
Now, imagine if you have to be a scribe and you have to-- this would occupy a text of a thousand volumes, each a thousand pages long.
And at the end of the process, you've only made three letters mistakes.
Now, how do we actually get to the idea of gene targeting?
Volker hinted at this and next to be on the lab, we started on this word shortly after in the late '70s.
Axel-Wigler had just come out with a paper showing that if you take DNA and you make it precipitate with calcium phosphate, and you dump it on top of cells, the cells ate it, phagocytized it, and a small fraction of that DNA would get into the nucleus and be functional.
For example, if the cells were thymine and kinase minus it's provide with the DNA sequence for making a thymine and kinase gene, one in a billion cells would then acquire that DNA functional form.
So, when that paper came out, so this was 1977, I thought, well, maybe the reason that it's not efficient is simply the most of the DNA is going to the lysosomes, being degraded and therefore never getting to the nucleus.
So if we actually took a little-- a hypodermic needle, the really small one and filled it with DNA then directed it into the nucleus, maybe it would work much more quickly and this is the apparatus we put together, we simply copying essentially, a neurobiologist who's right next door, never using this equipment for measuring concurrence between cells and inside and outside the cell.
And what we simply change is simply that-- put pressure and then allow us to inject DNA.
And remarkably, the solution that the DNA is-- has a different refracted index.
So, when we got into the nucleus, we could actually see the solution, spread in the nucleus, and we could utilize that to tell us how much volume we were injecting into the cell.
What's the [inaudible] a couple few percent.
So it's very accurate 'cause the nucleus were less constant where it given self-time.
So this then [inaudible] if we know the concentration allowed us to quantitatively put molecules, how many molecules want in any given cells.
And amazingly, it worked.
Instead of now having this frequency being one in a million, the frequency was now one in three.
And this led essentially right away to making transgenic mice which the DNA was inserted randomly within the nucleus.
But the observation that I found interesting is the following one.
So, we could inject as many molecules if we wanted of a given time.
And what we found is as predicted from Wigler and Axel, they would go randomly into a position in the chromosome.
But what's amazing, they were all lined up in the same direction.
DNA has a direction, spread left to right as you would a text for example.
Okay, so here we're introducing a bunch of molecules in the same sequence and then we look at it and then the random locations are all lined up.
And we could inject a thousand molecules and all of them would be what we call head-to-tail concatemer.
This cannot happen randomly.
That's impossible.
So there were two principal methods that this could have be happening.
One was it was a synthetic machine, okay.
Imagine what we use to call a rolling circle model DNA replication.
Sort of [inaudible] machine where it would act-- one molecule would act as a template and then it was turned up more and more copies of itself until you have this whole [inaudible], a head-to-tail concatemer.
So that's one type of model.
The other possibility was by homologous recombination which Book just mentioned.
And that's shown here.
So this is the same sequence, I've colored it different color so you could follow them.
And what homologous recombination does is perform an exchange between these two molecules.
Breakage and then joining but it's done perfectly.
So if you have text along this line and this line and it's the same, after recombination that text has been altered.
So to the nucleotide, it's maintained and that's what homologous recombination means that you have to have all the genes [inaudible] with each other and then you have an exchange.
Now, the reason this was surprising and not only surprising but very efficient, remember, I told you a thousand molecules and they're all lined up.
So the reason that was surprising was that people knew about homologous recombination but they all thought it was about sex, okay.
That is-- it's a way-- instead of you getting a whole chromosome from your father or mother, it would be always a mixture of the two chromosomes that are present in that parent and the other and they had exchanged each other by homologous recombination.
So that makes a repertoire of different alleles that you're handing on to your children be very different from sibling to sibling, okay.
So, you know, the good thing about it is that your siblings look a little bit like you but not too much.
[laughter] So this allows essentially a way of spreading out a number of different alleles that are present.
And this is what everybody thought homologous recombination was about, but we were working with fibroblasts.
That's connective cells in the skin.
So this is a somatic cell.
So it had nothing to do with sex and it said that somatic cells presumably any somatic cell has a machinery to be able to do homologous recombination and to do it efficiently, okay?
So that right away said, "Wow!
We could utilize this machine to do gene targeting." Right away, that was obvious.
Could we present the DNA in such a way that it would utilize it as a method essentially of incorporating itself by homologous recombination to change the genome of that particular cell.
So this is simply an illustration of how you could generate.
For example, here, that linear molecule, here's a circular and you exchange across [inaudible] and see you could get perfect repeats and you could do this over and over again and that would give you the peak concatemer.
And this is now simply a similar transition except it requires two exchanges to do what we call gene targeting.
So this represents your favorite gene and the asterisk represents the modification you've done to that gene.
And what I want to stress is that that modification can be anything.
It can be single base pair chain, addition, deletion or substitution or the addition, deletion, substitutions of thousands of thousands of nucleotides and now we're actually trying to extend that to millions upon millions of base pairs.
That technology doesn't exist yet.
So-- And we also decided, we wanted to do it in a mouse.
Okay, so these are our patients.
And unfortunately, I told you that it works, we get gene targeting.
I should point out-- give you a little bit of history.
In 1980, when we first started thinking about this, we saw that, you know, we could get recombination essentially between two pieces of exogenous DNA then we extrapolate and so, well, why not do it between exogenous DNA and this the same sequence in a chromosome.
Okay, we submit a graph and-- in 1980 and they said, "No go.
Impossible." Okay, so-- and the reason for relaying is simply, if you had an idea and you're convinced about it, go with it anyway.
So what we did is utilize money from another grant and to continue the idea.
[laughter] Four years later, we have some success, we sent it back to the exact same study subject.
The pink sheet which is the evaluation sheet they sent you started out by saying, "We're glad you didn't follow our advice." [laughter] So I think, you know, that's the persistent aspect of biology.
But coming back to these mice, we know we wanted to do-- work in the mice and at the time, we also knew that the targeted frequency was actually very low.
It was going to be roughly in the one to ten thousand, one in a million events.
Okay, so, that meant we will not going to be able to do it in the zygote itself, you know, or in a oocyte, you know, anything that requires single injections and then making a mouse out of that system.
So we needed a culture system to be able to do that and those cells didn't exist.
ES cells did not exist at that time.
And instead what existed what EC cells, also known as embryonic carcinoma cells.
And these cells are derived from a-- an embryonic carcinoma cell which is sort of a mixture, a bag of many different types of cells.
I mean, they're actually fairly disgusting.
And-- But [inaudible] embedded in that are cells that are actually capable of giving rise to all of the tissues.
I mean, what you see in this messes is teeth and hair and muscle, you know, anything you can imagine but all jumbled up into one mixture.
But in those were EC cells.
And EC cells were shown to be pluripotent both in vitro and also actually could make mice.
But they didn't contribute to the jumbling.
So that meant that in essence we were at dead-end in the sense that you could make mice, but then you have to make a mouse over and over again if you wanted the same mouse over and over again rather than being to able to breed those mice to then get whatever change generated, propagated in the progeny.
So, first question you might ask is now why did you like these beasts?
They're small, they're fuzzy, white mouse, and we have a common set of genes.
99.9 percents of genes in terms of gene content are the same in humans and mice.
And so, we also have a common developmental plan, plan in the sense that they're mammals.
So, plumbing is identical, the physiology is identical, the nervous systems.
In fact when we started this work, there were no atlases with respect to the brain atlases.
And what we utilize were actually human atlases to tell us where we were in the mouse brain.
It's-- There's that much continuity to the systems so we could recognize exact whether we were in the hippocampus, whatever, okay.
But most importantly, we can manipulate the mouse genome in any conceivable matter through gene targeting.
So that was the mouse.
And at the time, ES cells didn't exist.
And I'll relay the story again because now often people say science is extremely competitive.
Here's an example whereby working together, all of us profited, well, all of us-- this-- all of us meaning, Martin Evans and Oliver Smithies and myself with the principle labs at that time working on this particular problem.
So-- And then, this simply says, you know, you have the same set of genes that whatever we find out in mice is also likely to be true in humans.
So, at the time, all of a sudden I heard-- I was going to a lot of meetings about EC cells, embryonic carcinoma cells.
And the more I went to that, the more depressed I got, simply because there was one report that they had gotten the germline and then few months later, it turned out that it couldn't be possibly be correct simply by looking at the genotype of the outcomes.
So something had gone wrong.
But-- So it doesn't-- it didn't look like a germline transmission was possible using EC cells.
And what Martin-- I'd heard a rumor that Martin Evans was looking for EC cells but starting with real mice.
Looking-- Starting with embryos and trying to isolate from the embryo cells were very similar to EC cells.
And what the time we're calling EK cells but now are called ES cells.
So I heard this rumor and so I actually called them up and he said, "Yes, we're working on this.
It looks like it's beginning to work." And so I asked him could I come to his lab and he said sure, come in to his lab.
My wife and I went there, spent several weeks, and simply learning about how to make ES cells and then how to make-- use it to that and make mice.
So here's an example essentially of cooperativity benefiting ourselves as well as science.
So, here is, again, the gene targeting, cancer-- rendition.
And so here you isolate that gene, modified in some way, put it back into the cell.
It combines with the machine.
It's a very complicated machine that's actually fascinating.
It contains about 25 different proteins then searches the entire genome and thinks about 30 minutes to find that cognate sequence.
The criticism from the granting committee was that that would never happen.
And here it just happens, zip, and then really amazing how quickly.
And it actually occurs so quickly that you can calculate it.
It can be simply three-dimensional diffusion.
It's actually has to be one-dimensional.
Hops on the chromosome and rips through that chromosome, looks for the sequence, if not hops on to another one, and so on.
So I'm going to give you an example.
So here are the ES cells.
These are mouse ES cells.
And these are being grown essentially on [inaudible] there which is supplying the properties to the making this ES cells.
And these again are remarkable cells.
They maintain their pluripotencies.
We can grow them forever.
We could put them in a freezer.
We could pull it back out of the freezer and they maintain the pluripotency.
Meaning that they're reintroduced in an embryo, they contribute to all the tissues of the mouse and most importantly, they contribute to the germline.
This is again the same machine I just showed you or we could use the exact same machine to introduce ES cells back into an embryo.
Preimplantation embryo, here it shows that, so here's the preimplantation embryo as being held in one pipette and this pipette has the ES cells which we've now modified genetically using gene targeting.
Here you can see the cells getting inside then they mixed with these cells.
These are the inner mass cells which are responsible making the embryo and then participating and making all the tissues of the mouse including the germline.
So here's the [inaudible] and here's the ES cells modified, preimplantation embryo.
Once you've done this experiment, you make a little slit in the back that exposes the uterus and then you can introduce that embryo back in.
And then allow its continued progression to make a mouse.
And if you choose [inaudible] distinguish the source of the ES cells from the source of the recipient blastocyst then except one being brown, the other being black then you get out chimeric mice, these mice have [inaudible] parents.
And then by appropriate [inaudible] you can get a pure mice with derived completely from the ES cells.
In this case, big brown for a goody.
So let me give you an example of some the [inaudible] experiments what can do.
So in this particular experiment what we're doing is taking advantage of a gene from E.
coli and gene from jellyfish, one that in the presence of the appropriate sub-state turns blue, the other simply fluoresce as green.
And so if you introduce this gene or those two genes separately into the same gene then you can see where that gene is functioning.
So wherever those-- that gene is functioning those cells are blue or fluorescent.
And you can see that Hoxc13 is important for making fiber cell.
This gene is also important in making males.
So here we're introducing beta-galactosidase into the Hoxc13.
And then you get blue males or green males and you can ask what is the function because if you introduce one gene into the middle of another gene, it activates this gene and you can ask what happens.
Here're the experiments, plus-plus is two normal copies of that Hoxc13; plus-minus is one defective copy; and then 25 percent of the progeny will have two minuses and what you can see is alopecia.
This mouse is bald.
So you know that this gene is important for making hair.
It also has defective nails.
And so here we saw normal nails and here's defective nail as a consequence of injecting either the GFP or beta-galactosidase in the middle of those gene.
We can't go to the human population and ask other people with alopecia and defective nails and there are.
And then you could look at their DNA and they have defects in Hoxc13.
So this tells you in essence that whatever we're seeing in the mouse is like be true also in humans.
And this-- And this scenario done over and over and over again thousand upon thousands of times.
So now let me turn to more general question.
Now, what motivates a scientist?
I think there are three reasons.
I think, one, is simply just discovery, the joy of seeing something that's never been seen before.
The other is utility that is we hope that whatever reason-- seeing is actually useful to society and in the case of biological science is to medicine.
And third is science is a unique community.
It is-- has [inaudible] any country can have terrific science.
It's always build up upon the past.
So we're always-- we're never doing science in the vacuum, we're always adding to what other people have already done.
So it's a community in the sense that's always progressing and it's open to anybody.
And I think that's quire remarkably in terms of, you know, communities in general that nothing like it exist in the world except through, essentially, science.
And it's also-- we do arrive at truths.
I mean truths can change.
The experiments can change because the paradigm of science is that it's reproducible.
If I do an experiment and have to describe it well enough to be significant so that another lab can repeat it, okay.
And in that way purifies itself.
That's the component built into the system to make sure that whatever information [inaudible] reading is of value.
Okay. So now we come to the more embarrassing parts.
And that's early influences.
And early influences always takes you to your parents.
So this is a picture of my mother.
I only have a few photographs of my mother.
Here she is at age 19.
Her passion was languages and poetry.
And this is her at age 30, a few years later.
Now, she, as I mentioned is a poet.
Let me take you back a little further to grandparents.
Her mother was actually an artist, a painter and she grew up in Oregon and at age about 17.
She decided she wanted to become a painter and she wanted to go to Florence to learn how to paint.
So she packed up her [inaudible] trunks with her other, left her husband and went to Florence and started painting.
She became a very good painter.
Let me show you a couple of pictures because they also illustrated their life.
They grew up in a village of Florence.
This is my mother.
These are my two uncles and this is the olive groves Florence.
And what you can see is that there's strong influence of impressionist paintings.
They lived in a beautiful villa.
They had cooks.
They had cleaners.
They had gardeners.
They had everything, tutors in languages, history, in archaeology, and the science and so on.
Life is pretty splendid.
This is a picture of my mother at age 8 and my uncle Edward at age 6.
Again, in the-- in Florence, the-- in the villa in Florence.
Then first of all, war came and the father was actually killed.
And so then my grandmother had to then raise the three children on her self.
What she did was to paint and then converted the villa essentially into a finishing school for young women mostly from United States.
So, an idealic upbringing, and then my mother became-- her forte was poetry and languages.
She went to the Sorbonne in Paris, became a lecturer and then became associated with a movement known as the Bohemians.
And these were artists, meant most of the writers and poets who were essentially fighting fascism, fighting nazism using [inaudible] theory.
And then in 1937, she moved to Tirol here.
It's just north of Bolzano, just above the Bolzano.
This is the chalet that she was renting.
This is my mother right here and I happened to be inside there somewhere at this age.
And she knew that her time is limited.
She, because of her political activities, what she did was she knew eventually she is going to be picked up so she actually sold all her possessions and gave the money to a farming family and said that if I don't get picked up, please take care of my son.
And in the spring of 1941, the Gestapo did come and they did take her away and in turn-- she was entered in a concentration camp.
We don't know exactly where, our best guess is Dachau 'cause the best information we have was that during this period, she was near Munich.
Dachau was built essentially for political prisoners.
So this is simply-- oops, go back.
No, okay. Now, that was simply to illustrate-- this was actually a picture taken in Dachau, took my daughter there when she was a young girl just to illustrate what horrendous things are going on at that time.
Now, this is actually a picture of a farm that I was-- rubbish, so this is at age 3-1/2, I move to this.
And this is also essentially as a consequence of Nobel Prize.
They found where the farm was and they took me there and the same family that owned it when I was there still owned it and they-- this building was actually taken down and a new one has replaced it.
But I was able to tell them where the chicken house was and so on and it's quite remarkable to go back to those footsteps.
After one year [inaudible] to me, I don't know how, all the money that my mother had given to his family actually disappeared.
And as a consequence then I was put on the road and I started simply marching southward, during this period.
And so-- And, you know, I was living essentially in the streets, sometimes I'm being picked up and not thrown into an orphanage that was worse than being in the streets.
I'd run away then travel to the next city and so on.
And this is existence actually from years 4-1/2 all the way to 9.
And that in 1945, Munich was liberated and my mother started looking for me.
It's the spring of 1945 and then essentially October of 1946, a year and a half later, approximately, she did find me.
And I happen to be in a hospital and everyone in that hospital of that time was there as a consequence of either having typhoid, malnutrition or both.
And the perspective-- the possibility of those children ever leaving was almost impossible 'cause they haven't left no food essentially.
We were living on a daily cup of Chicory coffee and a piece of old bread that was really tough to even be able to break with your teeth.
So that was the nutrition that we were receiving at that time.
She arrived one day, she did illustrate-- she had [inaudible] dramatic.
She arrived on my birthday and I didn't recognize her.
I mean, the five years completely changed her physically, psychologically and was not recognizable.
But I knew that wherever she was going was better than where I was and I'm certainly going to go with her.
She got me-- the reason that I was in the hospital-- I mean, I look at-- you know, I was very good now getting out of places.
But what they did was take away all your clothes.
And so, you know, and you had to have some clothes to be able to run away.
[laughter] So that-- And at that time, then my brother-- her brother, my uncle, was living in United States.
He sent money for a boat and then two weeks later we're in the United States.
And literally, what people expected, what I expected was streets essentially laid with gold.
This is the perception of all the people in Europe at that time.
And what I found was actually much more and that is opportunity and I think that's the one thing that I do want to get across, is that we are really blessed with opportunity.
This is my uncle.
I told you he was a physicist.
He is a very good physicist.
He was one of the first people to figure out how to like medically be able to focus electrons and made actually the first electron microscope.
Here, he's working on something that he was not proud of, this is television.
And television was not allowed in our house ever.
And he was instrumental in developing both black and white and colored television.
But what he have done in United States was to set up a commune.
This is very similar to Kibbutz.
Everything is only common and everything was made essentially by the hands of the people living there.
Still exists, the Pennsylvanians [inaudible] it's a family about-- there are 65 families, living community.
So imagine going from the streets of Italy to a commute, okay, from a completely asocial environment to an extremely social environment where everybody knew everything.
So that's where I was brought up, but it was fantastic for kids 'cause you have 65 parents and it was enormous amounts of activities both in the arts and sciences and religion.
Quaker-- It was a Quaker community in the sense it was started by Quakers but it was open to all religions and all races.
So this is in the '40s.
And now, you know, you could imagine again what outside communities were thinking it was going on in that particular community.
So it's enormous contrast.
This is actually a picture, this I my daughter feeding the geese up in [inaudible].
This is myself about six months after coming to United States.
Had never been to school, didn't know how to speak English and was immersed essentially.
I arrived on a Sunday, Monday I went to school.
[laughter] They are maturely thought-- I was nine years old, you must be a third grader.
I spend the third grade.
[laugher] Then public school system like the third grade teacher I had was fantastic.
She was amazing woman.
And what she did was simply-- she had [inaudible], I mean, this-- you can get rolls of, you can, you know, roll across this whole stage essentially with one huge piece of [inaudible].
And what she said is make them your own and they were studying Holland at that time and so I made, painted a way, people would touch costumes, cheese and boats and you name it.
And so that didn't require communication.
It didn't require communication.
And that allowed me to simulate into the class.
This is actually the high school I went, to which a Quaker school and it's a very good school.
It's called George School.
It's just outside of Philadelphia.
And again, [inaudible] with community with respect to teaching, particularly compared to with the public school of that-- in that area.
This is actually George School, again is Quaker-- Quakers-- they don't believe in frills but they believe in service and they believe in people to solve their problems.
And one of the things I did during this period of time actually two summers is work at Mexico.
This is [inaudible] this guy here, he's the guy that doesn't have any-- his teeth are missing.
It was an accident on wrestling.
[laugher] But it was again an enormous experience in terms of working with people in the tremendous community, in Mexico in this case.
After George School, I went to Antioch College.
And Antioch College is a liberal arts college in Ohio.
And what's distinct about it is that they have this work study program.
You work a [inaudible] and you study [inaudible].
Work a [inaudible] you study [inaudible].
And those jobs are all over the country and they're related to whatever you're doing.
I was doing lab jobs, so then I was going to lab jobs here and there and every where.
So by the time you finish this and have to graduate you've already have-- it's as five-year program, you've had two and half years of experience in a particular discipline.
And at that point you know whether you like it or not like it.
And so it allows you to really get into that area and, you know, it's-- we-- this is in the late '50s-- now early '60s, this is just at the time when molecular biology was being born.
And it was a remarkable community again because they had the influx of people from chemistry, physics, biology, from genetics, all of the people from very different disciplines but working at a common end, and a new way of thinking of how to solve very complicated problems in biology.
And the premises was it's simply that no-- one is physical approach with work and we could work with simple organism, viruses, bacteria, and then whatever we were learning would that be applicable also to much more complex organisms.
This is a-- then after [inaudible] I went around to different towns, looking at Harvard, MIT, Caltech, and I interviewed with this character, Jim Watson, and I ask him, you know where-- why should I go to Harvard?
And he looked at me, he says: "You'd be fucking crazy going anywhere else." [laughter] That was very persuasive.
[laughter] So I ended up in Jim's laboratory.
So he had-- what you learn from Jim is how to do science.
How essentially go into a field and start seeing what questions need to be asked.
You know, one of the things he continually told me was simply, "Don't ask simple question because you're likely going to get simple answers".
Okay, simple [inaudible] to the big question.
And he's a tremendous mind.
We also had at the same time Wally Gilbert who was a physicist and the two complimented each other enormously because they thought completely different.
Jim Watson is extremely intuitive.
He simply knows how biologists like to work.
Watson-- I mean, Gilbert on the other hand, from physics is very quantitative.
Watson is not at all quantitative, terrible experimenter, but intuitive.
[laughter] And actually Wally was actually a very good experimenter.
So having both of them in the same lab was, you know, tremendous, and they also, I mean, where-- juxtaposition, was, you know, you wrote a paper, you presented to one, they shred it all up and then you give it to the other they shredded all up, because they're looking at the world from very different eyes.
But through that synthesis, you got a lot more than have either been associated with one or the other.
But-- And then after graduate school and then I went over to Harvard medical school, Harvard medical school was terrific.
But-- And I was there for four years then had moved right through the ranks, but something was missing.
And there were couple of things.
One is that there was a very bad collection of people.
They were an excellent scientist but simply did not get along.
And so-- you know, so it didn't have any symmetry, everybody was a islet upon them-- among themselves.
And the other aspect is that everyday we were asked, "What's new?" So if you're asked that everyday, pretty soon you want to work on projects which have in a few days you have results.
So many interesting projects are actually long time.
They require a long period of time to develop.
And I knew that I wanted to work on gene targeting and didn't know exactly how, but I knew sort of how to sneak in to it.
But I knew and also is going to take a long time, and it took ten years.
Okay. That I don't think would have ever happened at Harvard medical school.
But in Utah, this-- and the person that got me there is this, Gordon Lark, he's also an outstanding scientist.
And he had a good sense essentially on people and bringing people together from-- that would synergize, that would want to work together, to enjoy themselves.
We're science because it's fun.
If it's not fun then, you know, go into something else because you'll make a lot more money on something-- doing something else.
But you know the challenge inside is simply that is never been boring, it's always changing.
And every time we're all there, we're simply just always solving puzzles and that's what makes it exiting.
And Gordon was very seductive and told me, you know, it took about two years to convince me to come to Utah.
And then, you know, the Harvard community said, "You're going where?" [laughter] And-- But I did actually get counsel from Jim Watson, and he said: "Go for it, you can do good science anywhere." One of the reasons I want to Utah is this is what we look at the window of our house.
This is in the spring time.
Here it is in the fall.
And here it is in the winter.
So it can look by [inaudible] spaces, and that liberates the mind.
Here is my wife and newly born daughter, just when she was born.
So this is-- and this is her a couple years later, and this is our house in the back.
The out house is over here.
[laughter] There is no heating in this house, we simply have a wood stove.
It's quite primitive.
It's completely isolated, but it's beautiful.
And I think, you know, having space, having air, being in contact with nature, I think does free the mind.
So finally let me give you just a quick trip through a stop.
These are the players in 2007, so these are two physicists.
This is from France.
This one in Germany, the chemist.
These three characters I think you might know Oliver Smithies and Martin Evans-- Sir Martin Evans, and these two are economists.
Here's a closer look at the trio and, you know, I want to reemphasize that during this whole time we would competitor, we're sharing reagents, talking to each other all the time, meetings and meetings and sharing.
And I think we had a community and as a consequence, I think, all of us did much better and had [inaudible].
You go to Stockholm in December, okay, you have about four hours of light.
[laughter] It's cold, but it's extremely beautiful.
And the reason Stockholm was beautiful is because it's inundated by the ocean.
Where ever you are you're always seeing water.
You're always looking at the boats there.
So I have lots pictures of boats.
Boats, boats and swan.
[laughter] This is the hotel we stayed at.
It's again on the water, the Grand Hotel.
When you arrive, they pick you up in this huge limousine-- a black limousine and that person, the driver associate with you, the party last 12 days.
You have to brace yourself.
[laughter] You arrive at the hotel and there streams of people there waiting to get autographs.
Three days later, Bruce Springsteen comes to the hotel.
[laughter] That whole crowed disappeared.
It humbles you.
It shows you were scientists stand [inaudible].
This is my Swedish wife.
He's responsible for this-- He's from the-- He's apartment [inaudible] and is responsible for everything you do.
Again, 12 days, starting at 8 a.m. and ending at 3 a.m. So-- And he tells you how to dress, how to behave yourself, how to behave in front of the prince, princess, the king, and the queen and so and so on.
So he is indispensable.
Fantastic person and that's his wife.
This is during the ceremony.
So here is [inaudible] the family.
Here are the Prize winners and these-- the 50 people they're about to be choosing the Nobel Prize.
It's very similar to how a pope is decided.
[laughter] There's a huge table, a round table, they put me to that room.
They close the door and not allowed to leave until they reach a consensus.
It's not a vote.
It's not a majority.
They have to reach a consensus and they can't leave until they've chosen who it is for that particular year.
So there're these people.
Now, how do you behave?
These are flowers.
[laughters] Might ask where the flowers are coming from?
Italy. They're all shipped in for that day.
There're flowers everywhere.
It's magnificent.
This is the royal family.
And the way you behave is simply look at the king.
Whatever he's doing, you do.
So if he stands up, you stand up.
If he sits down, you sit down.
[laughter] It's quite formal but very predictable.
[laughter] Here are the pictures of the laureates.
I'll show you closer pictures, very funny.
[laughter] This is receiving the actual prize and you have to remember which hand is which.
Shake with the right one.
Get the prize with the other and so on.
Practice that and then here is again a person of responsible for our existence for those 12 days.
This is my daughter.
This is Gordon Lark and his wife at the ceremonies.
This is procession to the dinner.
This is very cordial dinner, 15,000-- 1500 guests.
And it's not-- you know, those-- every body is served within three minutes.
I mean, it's amazing.
They practice it and practice it just so they could-- they have to march down the stairs, get to the tables, and present you with the dinner within three minutes.
There are all sorts of plays, dances going on at that time.
This is the next queen.
This is Princess Victoria at the dinner table.
And then here, the dessert is coming in.
[laughter] And it has these screaming fireworks coming up which are changing color.
[laughter] So when it rise, it's red, okay.
And it's gone-- being one is gone through white, red, blue and yellow and so on.
And then, boom, red.
Lots of dancing, every night they just dancing, that's why the 3 a.m. curfew and-- this was very special.
Sweden has had an influx of enormous numbers of people from other countries.
And just fair to be-- so they built these communities [inaudible] with these-- harmonizes with, you know, that house millions of people.
And they have schools where literally in a given class will have 20 different languages.
So they have to maintain their own language and learn Swedish and English.
And this was [inaudible] day.
Usually these are actually lit on fire with the real candles.
This one is an electric and then these kids are singing and it was remarkably experience going to these schools with so many different people in a given classroom of different nationalities.
Things happen also as a consequence of Nobel Prize that you completely unexpected.
As a consequence of the people putting newspapers [inaudible], you know, these people won the Nobel Prize and so on.
This person [inaudible] look at it and said that has to be my brother.
I never-- I didn't know she existed.
She turns out to be a half-sister.
She's two years younger than I was and she was given up for adoption about-- less than a year after she was born to France because my mother knew she was going to be taken away.
She read a newspaper.
She thought she knew about us.
She thought I was dead.
She thought that my mother was dead.
And then she said, you know, so she communicated with us and I had the opportunity to go meet her.
And she's a terrific woman as a sister should be.
That was quite a surprise, very special surprise.
So I'll stop there and open it up for questions and thank you for your patience and I'd be happy to address any questions that happens to popped in your mind.
Thank you.
[ Applause ] So as a bit of advertising, tomorrow, I'll be giving you a scientific lecture on the work that we are currently involved in.
So any questions?
Don't feel shy.
I tell my students if you have a question, there are ten other people that have a similar question, so you're doing them an enormous [inaudible]-- whatever.
[laughter] Yes?
[Inaudible Remark] Yeah, I think, you know-- the question is what specific advice about working with competitors.
My own view is that if I can talk you into working on that particular problem and I'm working on it, that's fantastic, okay.
At least now there're two people working on it, okay.
So it makes more interest.
And each person looking at-- you know, no matter what, we're working on it, we're looking at it from different vantage points and we have different interests, okay.
I'll emphasize A, you will emphasize B, okay.
So I think you almost always win by actually sharing expertise as opposed to keeping it secret from each other and then trying to race with each other as well.
And I'm going to should point out that Jim was the classic in terms of setting on competitions.
I mean, he had us-- in one class it was Gordon [inaudible], okay.
He was working on RNA phages.
He would actually not send anybody his RNA phage.
Crick actually got that RNA phage, [inaudible] working with by getting a letter, taking it, putting in broth and then isolating the virus from letter.
So he was the only that bounces that particular [inaudible].
We were actually working with a similar virus but completely different strain.
So here is-- but what Jim would tell us is he's about to just do what exactly what you're doing.
You better, you know, work like hell, okay.
Meanwhile, he was [inaudible] and it goes over and tells him what we're doing and what our results are [inaudible], but unbeknownst to us he's-- would set up the competition, but in actuality, he was actually sharing work but making us work like hell.
I don't recommend that.
I recommend [inaudible] sharing of information where you act-- and I think usually, you benefit.
I mean, it's rarely that you don't.
It's imply because, again, you know, the amount of-- right now we know is, you know, is not bad.
And what needs to be known is out there.
So is-- you know, there's an enormous amount to learn and in any given field doesn't matter, you know, how specific yet, you can always go deeper and different people would go deeper in different ways.
I'd be interested in A and you would be interested at B. Yes?
>> How do you keep up the relationship?
>> Boy, that's a tough.
How do you-- The question is how do you keep the [inaudible]?
I mean, that was one nice thing, you know, when we started, you know, there was only a handful of germs and that-- and you could keep with that.
Now, it's impossible.
I mean, you know, what I do is simply and have set of journals that I do go through rigorously, you know, but there only a handful, I can't handle that.
And, that the rest is always-- you know, then you write a paper, and then search it up, whatever that topic is, and to make sure that you aren't forgetting somebody.
So, it's very [inaudible] shot.
I mean, I think it is impossible right now to keep up with the [inaudible].
It's just enormous.
But, you know, the other thing that I do urge-- I mean, when I-- my own students, when I send them out to [inaudible], it's important for students to go out to meetings [inaudible], you know, that experience is-- pays off many, many times over.
But I send them to a meeting unrelated to what they're doing, simply because it's always a juxtaposition of the unexpected, that is interesting, bringing your own people to look at things from different vantage point.
If they're working on this particular problem, what in their technology might be useful to [inaudible] on?
Again, you'll always see that if you scamper further away, rather than if you're working on cancer, always go into cancer meetings.
You know, go completely separated, because I think, again, you know why molecular biology work, is because of the influx of different vantage points.
And, I think you can do that also simplify by attending different meetings.
And the other thing I do is actually purposely change fields, you know, working for about 10 years you work on this thing, then completely change in new area.
I anticipate where I'm going.
I often then teach course in that new field that makes me read the literature as much as I can, so I can then translate it to the students again.
And in the process now, you know, you have read new papers, new people.
You don't see this.
You know, if you're going to cancer meetings, pretty soon you always see the same people.
You jump a field, all of the sudden you don't recognize anybody, that forces you now to [inaudible] with other people, and working on new things.
And there's another advantage, and that is, when people are in the field, all of a sudden, they know what's important in that field, right, somebody comes in to this field, they could be living naive, they don't know this, they're-- you know.
And therefore, I might ask questions through my known naivety, that they may not be addressing and that way, we'll be able to contribute to a new area, which is being done, you know, by what particular people are working in that area.
So, I think-- and the other thing is to simply, you know, [inaudible], and that's all the simple thing.
It's always good to shape those things and see what happens.
[ Laughter ] [ Pause ] [ Inaudible Remarks ] When I-- When starting the lab-- what?
[Inaudible Remark] >> What-- So the question is do we know the implications of what we're doing?
Yes. I mean, I think we purposely went-- you know, once you recognize that a cell was able to do homologous recombination, we then, at that point said, we want to disrupt genes in mice.
Even though we didn't know how to get there, and even how to make to mice.
And how to make mice at that time didn't even exist in terms of ES cells, working existence that will give rise to the non-somatic tissue [inaudible].
So, I think nevertheless, you can envision that.
And that's what we did.
So, we had actually our direction.
We knew where to go.
We simply didn't know how to get there, and it took 10 years.
So, you know, what could come right away.
There were many ups and downs, you know.
And, I enjoy that kind of science.
I mean, I think-- you know, I look upon science sort of as a series of concentric circles.
The ones smallest around you are things that are immediately obvious, okay, and they need to be done, but you know exactly how to go and-- and then the circles further and further out are then populated by fewer and fewer people, and less and less obvious how tom get there and often required new technologies to get there.
And then finally, the last circle is science fiction.
And you don't want us to [inaudible] that line.
[laughter] You want to get as close as possible and because I think that's where the interesting phenomenons are going on.
Yes?
>> How large is your research group?
>> The question is, how large is my research group.
It's a steady-state.
It's been steady-state forever.
Forever is-- you know, when people ask how long I've been in Utah?
I said, "forever?" Meaning 30 years, that's a long time.
And, it's five graduate students and 10 postdocs.
And then we have support staff 'cause we have 20,000 patients, mice, [inaudible] so I have to have support staff to take care of the [inaudible].
But it's a manageable size, but-- and we can work on lots of different things.
>> Any other questions?
[Inaudible] one more time.
[ Applause ]
About the speaker
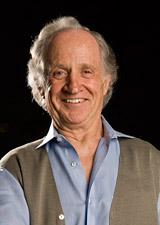
Mario Capecchi
Professor of Biology, University of Utah
Investigator of the HHMI
Nobel Prize in Physiology and Medicine (2007)
Born in Verona, Italy in 1937, he received his B.S. in chemistry and physics from Antioch College in 1961 and his Ph.D. in biophysics from Harvard University in 1967. He completed his thesis work under the guidance of Dr. James D. Watson. From 1967-69 he was a Junior Fellow of the Society of Fellows at Harvard University. In 1969 he became an Assistant Professor in the Department of Biochemistry, Harvard School of Medicine and was promoted to Associate Professor in 1971. In 1973 he joined the faculty at the University of Utah as a Professor of Biology. Since 1988 Dr. Capecchi has been an investigator of the Howard Hughes Medical Institute; since 1989, a Professor of Human Genetics at the University of Utah's School of Medicine; and since 1993, Distinguished Professor of Human Genetics and Biology.
Dr. Capecchi is best known for pioneering the technology of gene targeting in mouse embryo-derived stem (ES) cells that allows scientists to create mice with mutations in any desired gene by choosing which gene to mutate and how to mutate it. This gives the investigator virtually complete freedom in manipulating the DNA sequences in the genome of living mice, and allows detailed evaluation of any gene's function during its development or postdevelopmental phase. Research interests include the molecular genetic analysis of early mouse development, neural development in mammals, production of murine models of human genetic diseases, cancer and factors affecting life expectancy, homologous recombination and programmed genomic rearrangements in the mouse.
Dr. Capecchi is a member of the National Academy of Sciences (1991), the European Academy of Sciences (2002), and the American Academy of Arts and Sciences (2009). His prestigious awards include the Bristol-Myers Squibb· Award (1992), Gairdner Foundation International Award (1993), General Motors Corporation's Alfred P. Sloan Jr. Prize (1994), German Molecular Bioanalytics Prize, (1996), Kyoto Prize in Basic Sciences (1996), Baxter Award for Distinguished Research in the Biomedical Sciences (1998), Colby Presidential Endowed Chair (1999), Italian Premia Phoenix-Anni Verdi Award (2000), Spanish Jimenez-Diaz Prize (2001), Albert Lasker Award (2001), National Medal of Science (2001), John Scott Medal Award (2002), Massry Prize (2002), Pezcoller Foundation AACR International Award for Cancer Research (2003), Wolf Prize in Medicine (2002/03), March of Dimes Prize in Developmental Biology (2005), the Nobel Prize in Physiology and Medicine (2007) with Oliver Smithies and Martin Evans, member of the American Academy of Arts and Science (2009).