
Protein Interactions in Biological Complexity and Disease
Transcript
- >> Richard Cerione
It gives me great pleasure to introduce Tony Pawson to you today so this is his second Efraim Racker lecture.
Most of you, or many of you who were here last night, [inaudible] where we heard the Life Science Matters and some of you even found out how he got a Ph.D in two weeks by bureaucratic sleight of hand.
So I won't review for you again the many honors that Tony has won awards over the years, just going to tell you a little bit about the early history of his science.
So Tony was a Ph.D student at ICRF, Imperial Cancer Research Institute in London in right around 1980 or so, more than 25 years ago, working with a fellow named Allen Smith, who was a pioneer in vitro translation.
So those of you who think you can just call up at that time you could call up Promega Biotech and order your in vitro translation kit, it wasn't always that way.
Someone had to do that work and figure out how those things work.
So now of course, science is easier because companies sell things to us.
So anyway, so Tony went from the ICRF to UC-Berkeley to work with someone named Steve Martin, who was a virologist who worked on Rous sarcoma virus, chicken virus.
And that was a very exciting time, the early 1980s, the very late 1970s, an extremely exciting time because several things came together at the same time in understanding the emerging understanding of the molecular basis of cancer.
So at that time and in the previous roughly ten years people realized that retroviruses, which at that time were called RNA tumor viruses, caused cancers especially in mice and cats and a chicken, most of all in chickens.
So then in the very late '70s, around '79 or so, Mike Bishop and Harold Varmus in their labs and our post-ops discovered that the gene in Rous Sarcoma Virus that caused these cancers, then became known as the Src gene, that that was a mutated version of the cellular gene and that became a paradigm for oncogenesis, acute oncogenesis, by retroviruses.
And then in the subsequent years, the first two dozen or so cellular oncogenes that were found came from these retroviruses, especially the chicken virus.
At the same time, again in the very late '70s, phosphotyrosine was discovered, this kind of a magic signaling molecule or modification.
And then it turned out that the Src gene, this kind of a mother of all oncogenes, you could call that, was itself a tyrosine kinase.
And so that became again, became a paradigm.
So when Tony came to the lab -- I hope I have this at least roughly right -- or Steve Martin's lab -- and he started working on a virus that almost no one has heard of here except maybe David Salloway called Fujinami sarcoma virus, which is another chicken virus of the sort of -- probably a dozen chicken viruses have different oncogenes in them.
This virus was related to a sequence, DNA-sequencing was new then.
It had just been around about five years.
So the sequence showed that it was related to Src, also tyrosine kinase, and if you realize that there were regions of the primer sequence, the later were shown to be actually coding domains that were similar, and they named them sarcomology domains, so SH2 and SH3.
And again, that became a paradigm.
SH2 was sort of a granddaddy of the interaction domains, and now there are known, 25 years later, something like 100 is it, or 120 and still counting I guess, SH2 domains which grand foster tyrosine in the human genome.
These labs are pursuing this, and a lot of related studies having to do with signal transduction.
So Tony's a pioneer in signal transduction, especially as it relates to phosphotyrosine signaling and is a currently an international leader in this.
So he's going to tell us something about -- I don't know exactly what, but I bet that SH2 domains and phosphotyrosine will figure prominently in his talk.
So let's welcome Tony, and there's a reception afterwards, as you know.
[ Applause ]
- >> Anthony Pawson
Well, thanks for that.
It's a real pleasure to be here, a chance to give another talk, which I greatly appreciate.
And Booker was right.
There will be something there in the talk.
So let me start then by prefacing what I am going to say, by arguing and by the end of the talk I will convince you that there's some truth to this, that modular protein-protein interactions, let's say protein interactions mediated by folded domains and their recognition motifs, can provide a rather general mechanism to organize cell regulatory pathways and dynamic cellular responses, and certainly while this was initially recognized in the context of phosphotyrosine signaling. it, it has a sort of rather broad application to many different sorts of cellular regulatory pathways.
Secondly I sort of touch towards the end on the idea that these rather -- well, sort of theme of my talk I guess, will be that these rather simple protein interaction domains can nonetheless be exploited in the context of complex biological systems, and touch at the end on the idea that they can be appropriated by pathogenic proteins in the context of disease states.
So again, as Booker alluded to, the SH2 domains in the sense of prototype of folded interaction domains, so by interaction I mean something that can be obstructed from its host polypeptide or retain its binding activity and expressed in isolation, SH2 domains, then typically recognizing tyrosine-based motifs in a fashion that's contingent on their phosphorylation.
But there are now quite a large number of such interaction domains, so domains that recognize not phosphotyrosine for example, but motifs of the phosphorylates on serine and threonine which there are now a large number, FHA domains being one example.
Other domains, like I say, SH3 domains typically recognize short motifs.
They can be proline-based or some you may recognize, basic motifs, but usually not in a fashion that is directly dependent on post-translational modifications.
PDZ domains, I should say, [inaudible], recognize typically short motifs at the extreme C terminus, for instance, such as receptors, ion channels.
And then there are a number of domain families whose interactions handle the folded structure of both binding partners, for instance, death domains, form a hetero merit interactions that require death interactions require unfolding of both death domain -- both domains.
But so in addition to these peptide or protein recognition domains, there are a large family of additional interaction domains that recognize a diverse set of wide molecules, for example, nucleic acids on the one hand, phospholipids, Ptd, phosphoinositides, on the other there are domains that recognize small molecules, and metabolites and so forth.
So this is a rather sort of general feature I would argue, of the proteome.
And consistent with that idea about 70% of human proteins by our estimation have a sort of modular architecture in the sense that they have one or more recognizable domains, be they catalytic domains or interaction domains.
I sort of estimate there are about 100 classes of different modular sort of folded interaction domains of this sort, but each family could be found in many copies, so typically, each domain can be found in many copies in the human proteome, for example, so typically somewhere between 10 or 300 copies.
So if one looks at SH2 and SH3 domains, for example, in yeast there are about 30 SH3 domains, but about 10 times that number, around 300 on human SH3 domains.
And similarly for SH2 domains there's one protein involved in transcription regulation that has something that looks like an SH2 domain in yeast but it has no obvious phosphotyrosine binding activity, but in much [inaudible] approaches there are 120 transversion SH3 domains.
I'm not certain, they're still counting, because we think maybe there's something to be missed but we think into the 20s is the right number.
So you might wonder why this is such a common feature of proteins of multi-cellular animals, and I would argue, and this would be sort of a motif of the talk, that this sort of domain-based structure provides one mechanism by which new signaling [inaudible], new regulatory mechanisms, new biological functions can evolve.
And so as an example of that, an interaction domain, for example, could be incorporated in the course of evolution into many different types of proteins, and thereby confer recognition of a common feature, such as a site of posttranslational modification.
So consistent with that idea, there are many different sorts of domains that recognize distinct types of posttranslational modifications.
So there are domains such as SH2 domains that recognize phosphotyrosine-containing sites, there are several different sorts of domains that recognize phosphoserine, phosphothreonine-containing motifs, but there are domains that recognize -- such as chromodomains that recognize methylated lysines, domains like bromodomains that recognize acetylated sites, acetylated lysines.
There are different domains that recognize ubiquitinated sites, there are domains that recognize hydroxylated sites, hydroxylated proteins.
So a common feature of posttranslational modifications is that there, once a site is modified, they're recognized by faulted domains of one sort or another, and you can imagine, a straightforward way to confer an ability to recognize such a posttranslational modification would be to endow a pre-existing protein with one or other of these interaction domains.
So for example, in the context of phosphotyrosine signaling, growth factor receptors become autophosphorylated and interact with a number of targets that are quite diverse in their biological and biochemical functions, so they tend to regulate RasRap GDPases or control cytoskeletal, directly regulate gene expression, they move to the nucleus, they could regulate ubuquitination or phospholipid metabolism.
So but this diverse set of proteins have a common feature, which is the presence of one or two SH2 domains that endow them with this common ability to recognize phosphotyrosine-containing sites on activated receptors.
So the SH2 domain provides a sort of common device, if you will, to link these diverse cytoplasmic targets to activated receptor tyrosine kinases.
So that leads to the idea, then, that interaction domains could be combined in normal ways in the course of evolution to yield new signaling pathways.
So without going into sort of another story that I could tell you about this, but let me sort of briefly outline this sort of thinking, which is that in yeast, let's say, we have as I told you, multiple SH3 domains, which do important things, but there is no conventional tyrosine kinase in yeast and there are no phosphotyrosine binding SH2 domains, but as soon as one finds multi-cellular animals or indeed their immediate predecessors, such as Dictyostelium discoideum, or protozoans, Monosiga brevicollis, you find kinase as the phosphorylate tyrosine as a signaling device, and a code and appearance of SH2 domains, so presumably to, coupled in the sort of newly-created phosphotyrosine signal.
So you could imagine the SH2 domains could then become linked to pre-existing interactional catalytic domains to create new signaling pathways such as the sort of emergence of the adaptive proteins like this Grb2 Adaptor that could then be important for multi-cellular organization.
The question is whether that is actually a valid way of looking at things.
And we think it may be.
So the simple idea in signal translation is then that you have receptors at the cell's surface, they have to couple to aspects of the core cellular machinery, and one way they could do that is to exploit adaptive proteins composed exclusively of interaction domains with one domain that recognizes some feature of the activated receptor, such as a phosphorylated site and then additional domains that selectively interact with intracellular targets to yield any more or less selective output.
So the question would be are such adaptors actually important in directing signals that control mammalian, important mammalian cell fate, let's say.
So again, without going into the details over a number of years, we get more recently Janet Rossen's lab as well as many others, worked out a signaling pathway in the very early mouse embryo in which a receptor tyrosine kinase is likely the FGFR2 receptor, signals through this adaptor protein Grb2, small organization adaptor protein, again, likely we think based on genetic analysis in the mouse to the exchange of active Sos that then activates the rat's kinase pathway, and then downstream with this pathway is a transcription factor GATA6 that is activated by the pathway to induce primitive endoderm, is going to go into form or aspects of extra embryonic tissue, and also to suppress the expression of Nanog, another important transcription factor that gives rise to the epigloss, the beginnings of the embryo proper.
And just to show you sort of visually that there is likely important, here are embryos, embryonic day 3-1/2.
So this is before the actual manifestation event, so basically this is immediately preceding the appearance of primitive endoderm and epigloss.
So Gata6 is shown here in red, so this is under the positive control of that sort of Grb2 adaptive mediated pathway, Oct4 is another transcription factor shown in blue, and here is Nanog shown in red.
And you can see in embryos where we remove Grb2, there's a loss of expression of Gata6 and an expansion of Nanog.
So Grb2 is as important as playing a sort of important coupling role in controlling the signaling pathway that is important for this very early cell type decision in the formation of the mammalian embryo.
So you can see here that these Grb2- embryos then lack of what the catalytic Primitive Endoderm showed here by Primitive Endoderm marking.
And the wild type embryos which has been missing Grb2 mutant.
So that along with a lot of other data would be -- which I can discuss later if you're interested, consistent with the idea that these adaptors, whilst they're simple in design, might nonetheless be coupling events that play an important role in the organization of multicellular animals.
So then another issue is whether these broadly expressed adaptors could exert specific functions and specialized cell types, or rather whether they play important roles in various aspects of biology.
And to address that, we've looked at another class of these SH2/SH3 adaptors called Ncks, so there are two Nck genes in the mouse, so I'm trying to match it with the name NCk1 and Nck2.
And they, they appear, at least it's one of their functions, to signal specific phosphotyrosine signals to reorganization of the actin cytoskeleton, I don't have to convince you that that is one of their roles.
So Nck adaptors have this sort of domain organization of their C-terminus.
They have an SH2 domain as I'll show you likes to bind preferentially the phosphotyrosine sites followed by pYD [inaudible] forget the single-letter amino acid code.
And here are three internal SH3 domains that bind to conventional protein-rich sequences found on proteins that typically regulate the actin cytoskeleton, so for example, two well-known Nck binding partners in N-WASP.
It's sort of a modular protein that through the Oct.3 complex regulates branching actin polymerization in the serine 3 kinase that both through scaffolding interactions as well as its catalytic activity controls aspects of actinomycin dynamic counteractivity.
So Nck adaptors appear to be organized, as I said a couple phosphotyrosine signals to [inaudible] organization.
So what Nck might be actually doing in vertebrates or in the mouse, we freed up [inaudible] in the lab and inactivated the Nck gene of those mice were viable, inactivated the Nck2 gene which those mice were also viable.
Then we made the double mutants, the embryos died embryonic day 9-1/2, with really some clear detail on lacking defects in gastrulation.
So to get at this question of what Nck might be doing in specific tissues, we made a further mouse that is null for Nck1 and then has a conditional or flux allele of Nck2 which is functional until we express Cre recombinase in an appropriate tissue would then inactivate or at least suppress, the function of the Nck2 gene.
So that then allows us to analyze Nck function in specific tissues, and we've chosen initially two cells to actually to look at, cells called podocytes in the kidney, which I will show you in a moment, as well as neurons.
So what I hear you cry, are podocytes, a nephrologist -- actually I have no idea what a podocyte is.
But they are actually pretty amazing cells, so they're found in the glomeruli of the kidney, which is shown kind of in cartoon form here.
So there are capillaries that are lined by or formed by endothelial cells that essentially have holes in them, so fenestrated endothelium.
On top of that is a basal membrane, and then surrounding that is this podocyte, so the podocyte sort of sends out processes that wrap around the capillary as shown here.
So here's sort of looks like a creature from outer space, is the cell body of the podocyte that extends these primary microtubule based processes from which project actin-based secondary or foot processes, as they're called.
So these are purely actin-based structures and the foot processes from adjacent podocytes, you can see, second ones hanging down here, digitate, as they say, to form this sort of zipper-like structure, and there's a specialized cell junction that forms between these adjacent foot processes that is called a slit diaphragm, sort of essentially just a specialized sort of tight junction.
So the next slide I think is a cartoon of a section through to those adjacent foot processes, sort of shown here.
So here's this -- so the endothelial cell would be sort of down at the bottom, is a basement membrane, and here's the spec, is this foot process in the podocyte and here's this specialized tight junction.
So there's a critical adhesion molecule at the slit diaphragm called nephrin, which is mutated in some inherited forms of nephrotic syndrome, so if nephrin is nonfunctional, this slit diaphragm doesn't form.
And the slit diaphragm is thought to play an important role in filtration of the plasma, let's just say, in keeping high molecular weight molecules like proteins in circulation.
So if this type junction doesn't form properly then protein leaks into the urine and without a kidney transplant, those patients die.
So why would be interested in all this in the context of Nck?
And so it turns out that nephrin, which makes I should say, hemophilic interactions from adjacent podocytes, so has an extra set of a domain made of a region made of IgG repeats and then a cytoplasmate tear, which has been shown by Larry Holtzman and others to be tyrosine phosphorylated likely by Src family kinase such as Fin.
So over here it's simply an EM picture of what I just showed you in cartoon form.
So here's the fenestrated endothelium, this would be the blood vessel here, this is the basement membrane, and here are these foot processes from the podocyte, and here's my sort of artist's rendition of where nephrin would be located at these tight junctions.
So the question is -- I should say there is also some data in the literature that suggests that nephrin itself might be involved in organization of the actin cytoskeleton of these processes.
So in looking at the sequence of the tail of nephrin, we notice that it has three YDXV motifs, which as I told you, and we showed by looking at the binding of Nck SH2 domains to peptide arrays, so you can see that the Nck2 domain likes to bind phosphotyrosine followed by an aspartate and it doesn't really care, and then valine at the +3 position.
So these motifs in nephrin conform very nicely to this one that's being consensus which if phosphorylated, binds to the Nck SH2 domain.
So we then obtained a variety of biochemical data that I won't bore you with in detail, but which suggested that these sites were indeed phosphorylated by site family kinases and that when phosphorylated could bind the SH2 domain of the Nck adaptor.
I want to show you a little bit of data.
So in this sort of artificial setting, we expressed nephrin in 293 cells, we then expressed inactivated sites, and nephrin becomes tyrosine phosphorylated and co-precipitates with Nck with endogenous Nck.
Over here we've mutated those three tyrosines in those YDXV motifs to phenylalanine and you can see that the tyrosine phosphorylation events of nephrin has been significantly reduced and Nck is co-precipitating with nephrin.
We also isolated and purified glomeruli from the rat, in these ex vivo preparation nephrin is tyrosine phosphorylated and co-precipitates with Nck domain precipitation the other way around.
So it looks as though nephrin at least physiologically can be tyrosine phosphorylated and kind of associated with Nck.
So then we went back to mice, so remember that they're Nck1 null, they're fraught with Nck2, and then we expressed the Cre recombinase under the control of a podocyte-specific promoter the podocin promoter.
So then you can see that any animal with this genotype develops it, so this is on an analysis of protein in their urine, not a experiment I did myself, in fact done by a talented person, Nina Jones, who is -- anyway, so you can see that any animal with that genotype has massive amounts of protein in the urine, protein urea.
And if we look by EM, so here's an effectively wild type animal, this is -- so here's an endothelial cell, and these sort of little bumps which I hope you can see the foot processes from a podocyte surrounding the podocytes, surrounding the endothelial cells.
So here in the Nck1-deficient animals, here's an endothelial cell, here's the sort of cell body, if you will, of a podocyte, and so you see that there's a complete absence of these foot process structures.
In fact, we go back in embryonic department, they simply never seem to form.
So that suggests that Nck is selectively required for the formation of these actin-based foot process structure.
So our working model there is nephrin then Nck -- a couple tyrosines phosphorylated nephrin to reorganization of the actin, so we obviously then additional ways to test that idea.
And developed the following assays, so this was done by Nina Jones and Ivan Blasutig.
So pretty much everything we've done in associated with the next couple of slides, we've reproduced with full-length nephrin which we cross-linked with then antinephrin antibodies, but the safe ORC E's, we've in these experiments, adopted the following device where we make a chimera protein where the cytoplasmic region of nephrin is fused to the extra set of the main CD-16 and that simply allows us to readily cluster that chimera with anti-CD16 antibody and super cluster it with anti mouse Ig and when we do that, nephrin becomes tyrosine phosphorylated and that actually required cell kinase activity.
It's still entirely mysterious why clustering would induce tyrosine phosphorylation I should say.
And that leads to Nck recruitment, and the question is whether that has any effect on the actin cytoskeleton.
And indeed, it appears that it does, so in this experiment we take cells that lack both Nck1 and Nck2, so they were derived from entirely Nck1 null mice.
And we reintroduce a flag tag Nck2, nephrin, which is GFP tag and then we stain them for [inaudible].
I hope you can see that wherever nephrin is clustered in green, you can see these sort of - you see here these sort of comet tails with actin, sort of driven by these clustered nephrins, easy to see them.
This slide, this is sort of Christmasy slide.
So clustered nephrin, Nck and then just sort of comet tails of actin.
So here are just some few controls, so if we leave Nck out of those cells, you can obviously still cluster the nephrin but there's no obvious change in actin polymerization.
This is what I just showed you, that if we put in Nck2 it seems to be recruited to sites of nephrin clustering and coincidentally there's actin polymerization.
If we take a form of nephrin where we change those tyrosines when phosphorylines will recruit Nck to Phenylalanine, so can no longer biochemically at least recruit Nck, we can obviously still cluster that form of nephrin but Nck remains rather diffuse in the cytoplasm and there's no obvious change in actin polymerization.
So that then leads to the flying model that nephrin once tyrosine phosphorylate recruits Nck, that brings with it -- I will show you the data -- affected such as N-WASP, though we don't what the critical functional factors are.
And that can then lead to polymerization of the actin cytoskeleton in a fashion that the genetic data would suggest may be important, or is important for the formation of foot processes and thus for glomerular function in vivo.
And I should just make a point that obviously this is not functioning in isolation, so nephrin is known to bind the number of other signaling and regulatory proteins and there are other transmembrane proteins at this tight junction, including transmembrane proteins called nests, which have recently been shown by Larry Holtzman by Grb2, which can also interact with factors like in n-WASP, so there's clearly a really complex network, signaling network going on at this tight junction, which will be very interesting I think to dissect in the future.
One last slide to this little vignette is that Nina has recently succeeded in inactivating Nck, I won't go into the details of how that was done, sort of mass genetics, in the adults, so inactivated Ncks specifically in podocytes at six weeks of age.
And we sort of did this because the nephrologists I must say, when we came up with this original Nck difference, said, well, okay, may be important in development but then once the actin cytoskeleton is organized, you never [inaudible], it's sort of stuck there.
So it didn't -- actins dynamically, you think you still need some sort of signaling going on.
So and in fact that's what the data suggests.
So here's a control, here's a glomerulus, here are these sort of really nice sort of foot processes.
And here we inactivated Nck function at six weeks of age, post-natally.
You can see the glumerulus working after two weeks of following inactivation sort of becoming corrupted in the -- and the foot processes are breaking down.
So this would suggest that some form of this signaling is required continuously in a dynamic fashion to maintain these structures.
So the second sort of Nck story has to do with a possible role in formation of the nervous system, and particularly in axon guidance.
So we'd been interested over the years in the notion that axons, as they develop in the nervous system, feel out their environment with a growth cone which can then be either attracted or repelled by signals in the environment, so then we wanted to know whether these Nck adaptors might be important in this process of axon guidance, and we had some reasons that they might be because we and others had shown that a family of receptor tyrosine kinase is called EPH receptors, which I've shown diagrammatically over here, and their ligands confusingly called ephrins, nothing to do with nephrin, play an important role in this process of axon guidance in large part by mediating axonal repulsion.
And we had some data from some years ago, suggesting that Nck adaptors might function in a biochemical sense downstream Eph receptors.
So we made mice that lacked Nck1, conditional for Nck2, and that expressed Cre recombinase under the control of the nesting promoter, which is now broadly expressed neural and [inaudible] promoter.
So those mice had an interesting phenotype -- this is not the sort of experiment I ever envisioned doing -- but so these you may recognize are the hind paw prints of a mouse.
So the way you do this experiment is to steal your assistant's ink pad, get the mouse to run through the ink pad, run over a bit of paper, so here's the wild type mouse that has you and I on a good day is moving one leg after the other, and here's an Nck deficient mouse, and you can see that it has this sort of hopping or random like gait.
So why would that be interesting?
It's sort of interesting because it recapitulates the phenotype of mice that lack the Eph receptor, EphA4 So work from Klaus Kullander, Rubio Klein and others, have shown that EphA4 is expressed with a subset of spinal cord neurons, which are unable to cross the midline of the spinal cord beaus the midline expressed a strike of ephrin-B3, it's the repulsive ligand in EphA4.
So these neurons are constrained to one side of the spinal cord.
So in the absence of EphA4 or ephrin B3, there's an aberrant crossing of cortico-spinal track neurons as well as a set of interneurons that I'll discuss in a moment, called excitatory interneurons.
So interestingly, these EphA4 and Ephrin-B3 mice have the same sort of hopping phenotype.
So Jim Fawcett in the lab, who did these experiments, then looked to see what was happening to the cortico-spinal tract neurons in the absence of Nck, so these neurons originate in layer 5 of the cortex, they project down to the base of the brain and then they cross over, but once they cross over, as they project down the spinal cord, they stay on one side of the spinal cord because they're upheld by the midline.
So if we now take Nck deficient mice, we label them up here and the die then tracks down the cord of the cortico-spinal tract, so those neurons actually project okay until they get to the spinal cord.
And then you can see, if one looks at a one-type spinal cord, those neurons are restricted to one side but in the absence of Nck, they project aberrantly to the midline throughout the EphA4 deficient neurons.
So and in fact, if you look at a cross-section of the spinal cord an adult mass, so here's a structure in spinal cord called a dorsal funiculus, and that's where these cortico-spinal tract axons are running and if you look at an Nck deficient mouse, the dorsal funiculus is attenuated, which would be consistent with the notion that the proper spinal tract neurons are not projecting properly down the spinal cord.
So we then went to look at very early mice, and there's a reason to do this.
So these are P4 mice, so here's a wild type mouse that you can see is moving one leg after the other.
And here is an Nck deficient mouse, and you can see that it's as advertised, it's hopping.
So the reason for doing that is that at this age, those cortico-spinal tract axons that I just showed you have not yet projected to their targets in the spinal cord.
So at this age, that mere movement is dependent on a local circuit in the spinal cord called the central pattern generator.
And so the central pattern generator is a complex circuit that's only beginning to be understood, and I'll show you a very sort of simplified diagram here.
So there's a source of rhythmic activity, which is still somewhat mysterious, that activates excitatory interneurons that then are EphA4 positive.
So they're powered from the midline, they project to motor neurons which then fire.
But they also project to inhibitory interneurons that can cross over the midline, and so in principle, block firing on the other side of the spinal cord.
So this is argued to be the basis for the alternative firing of motor neurons on one side of the spinal cord, and then the other, which in turn is, it is a basic requirement for this sort of alternate linden.
So in the absence of EphA4 that has been proposed and experimentally tested that these excitatory interneurons can now cross over aberrantly so that you can get synchronous firing on both sides of the spinal cord.
So there's a sort of clever way to test this, which is to isolate the spinal cord from a young mouse, put on electrodes to record from the left and right side of the spinal cord, and then add neurotransmitters at which then stimulate this rhythmic activity, and you can see that if you record the left side fires and the right and the left side and the right side.
So really if you quantitated it, a perfectly sort of asynchronous firing on two sides of the spinal, so that's what's quantitated down here.
If you now do the same experiment but take this from Nck deficient mice, you can see that the firing has now become synchronous, so you've lost this asynchronous behavior, firing on the left and the right side becomes perfectly matched.
So we suggest, and we have some dye tracing studies consistent with this notion, and I'm going to show them to you, that in the absence of Nck, excitatory interneurons, even though they are EphA4 positive, can nor project again aberrantly across the spinal cord, to destroy the sort of fine structure of the central pattern generated circuit and thus result in a loss of asynchronous firing that's important for sort of walking behavior.
So these data I think at a minimum, suggests that Nck is required for signaling by axon guidance receptors in the spinal cord, and we propose that Nck might be coupling such guidance receptors to organization of the actin cytoskeleton that would have been in the growth, then lead to perfect projections of spinal cord neurons and thus the organization of neuronal circuits important for locomotion.
So as I alluded to, the phenotypes of these Nck deficient mice closely resemble those of -- I should have mentioned -- someone pointed out to me last night -- I should say that it's appropriate to talk about Eph receptors, Eph receptors and ephrin mutants.
So we speculate and this is very hypothetical in the working model that Nck might fly downstream of these Eph receptors in controlling the formation of neuronal circuits.
So I think these suggest that Nck can couple distinct receptors in different cell types to pathways that are likely important for complex cell morphologies, and the question we'd like to address is whether that signaling output is context dependent, that is to say, do different cell types have different downstream targets for different sets of targets for the Nck SH3 domains?
Do different receptors differ in their sort of potency, if you will, of Nck recruitment, so remember that nephrin has three sort of really good Nck binding sites, for example.
And how does the activation of parallel pathways influence the cellular response to phosphtyrosine and Nck signals, all of which we need to address?
So the last few slides let me speak to the issue of how these sorts of signaling adaptors and scaffolds, may be recruited by pathogenic proteins.
And one quick example involves this beast, enteropathogenic E. coli, which -- well, we're getting close to I discuss what they do, nasty as the case, cause of morbidity and mortality, especially in the developing world.
So this bacteria, when it attaches to cells, induces the formation of these sort of massive actin-based structures that [inaudible] call pedestals, and the bacteria attached to the top of those pedestals and never actually enters the cell.
So the bacteria introduces bacterium encoded proteins into the cell through a type 3 secretion method, but it sort of latches onto the tips of these structures that it itself induces in the cell, in a way that Brett Finlay and others, so we've collaborated with Brett Finlay on these experiments.
So Brett showed that the way that the bacterium EPEC does that, is to squirt into that host cell a bacterium coded protein called Tir, which adopts this horseshoe-shaped structure in the membrane of the host cell.
How that's integrated in the membrane, I think is mysterious.
And then it actually grabs onto the outside of the Tir protein, so it's sort of rather carries its own receptor around with it, sticks it into the host and then sort of grabs on, and as a consequence clusters the Tir protein, which then becomes tyrosine phosphorylated by Src kinase, abled of this tyrosine here, which we notice when we're looking at it with Brett, was in a YVXV motif, identical to one of those motifs found in nephrin.
And we were able to show that the SH2 domain of Nck binds with a rather tight affinity to that site, Nck, about 900 nanomolar.
This is the strongest Nck, SH2 site that's been identified.
Recruits N-WASP from the Arp2/3 complex, and one might pose that that was important for bacteria- induced actin reorganization, and in fact that turns out to be the case.
And if one looks here at some wild type cells -- these are massive rephotographs -- you can see EPEC, the bacterium in blue attaching to the cell wherever it attaches actin, which is now green, polymerized actin forms the site of bacterial attachment.
So here we've taken Nck null cells.
You can see that the bacteria can still attach, but there's a striking loss of their ability to polymerize actin in the absence of cellulary encoded Nck, then if we put Nck back into those cells then they'll recover their ability to polymerize actively.
So that suggests that this bacterially-encoded protein, by the acquisition of this Nck binding motif, exploits Nck, adapters to induce these actin-based cellular pedestals, sort of further argues that Tir, probably by process of convergent evolution, has acquired this nephrin-like YDEV motif which then recruits the Nck/SH2 domain.
And sort of consistent with that idea, we sort of recently did a simple-minded experiment where we took the bacterially encoded Tir protein in the context of the bacterium, mutated the tyrosine, which would normally be phosphorylated and would therefore bind Nck, and which results in a loss of Its ability to polymerize actin, and then just sort of stuck one of these nephrin YDXVs sites on the C-terminus, so we're basically giving the bacterium a sort of physiological site from nephrin.
And if we do that, probably this last lane is the last panels are the best ones to look at, basically where if you put in bacterium with a wild type Tir, you see that the bacterium attaches in blue.
There's entire phosphorylation actin in red, so you see this nice cluster.
That means that the bacterium is inducing tyrosine phosphorylation.
If we kill that tyrosine site in Tir-- actin phosphorylation rather is largely ablated, but now if we give it the site for this adhesive protein, nephrin, it now recovers its ability to polymerize actin so sort of a simple-minded experiment, consistent with the idea that the bacterium has learned how to mimic this signaling system based on this motif domain.
So the very last thing that I'll show you in a couple of slides involves the notion that oncogenic proteins, particularly oncogenic tyrosine kinases can exploit these sort of adaptors and docking proteins in their drive to induce malignant transformation.
So just summarizing data from a couple of papers that we published some time ago, and one just recently Rod Hardy, a student in the lab, showed that as in the slide, that when we mutate a docking protein called ShcA, which I will show you in a moment, that results in mutant phenotypes in the resulting mice, so phenotypes in the heart and in the skeletal muscle, particularly in skeletal muscle spindles, that closely resemble those resulting from the loss of the ErbB2 receptor tyrosine kinase, and in fact, ShcA has a phosphotyrosine recognition domain called PDD domain that is seen particularly well configured to bind to ErbB2, so it would be consistent that ShcA, which I showed you here, is a physiological scaffold downstream of the ErbB2 receptor, so ShcA has its internal, its simple phosphotyrosine binding or PTB domain, that like SH2 domains, will bind phosphotyrasine sites, though it does so in a different manner from SH2 domains, so that it has a C-terminal SH2 domain and then it has this sort of stuff in the middle, including two tyrosine-based motifs that become phosphorylated and recruit downstream adaptors like Grb2 to activate the Ras-MAP kinase and PI3 kinase pathways, as well as interacting with a number of the other polypeptides.
So if we wanted to test the model that ShcA might really be functioning downstream with ErbB2, particularly in the context of mammary carcinomas, so you may recall that ErbB2, by the way, is alternatively called Per2 , is overexpressed about 30% of human breast cancers, particularly those with a poor prognosis.
So mouse models of that human disease have been developed by Bill Mother, with whom I've collaborated.
So originally Bill developed a very sort of nice model where the mouse mammary tumor virus, LTR, is used to drive an activated form of ErbB2, which he and others have shown over a number of years, induces mammary carcinomas in mice.
What's sort of nice about this construct is that he's introduced an internal ribosome entry site created by the Cre recombinase, so that in the same cells in the mouse that are expressing the activated ErbB2, and which are becoming transformed, those expression of Cre recombinase, which could then delete a sequence that's surrounded lots of P sites resulting in a modification to that gene.
So here you can see this Cre recombinase in this system in viva, activating the expression beta-galactosidase in the mammary of the female.
So we then did the following experiment.
Where are control mice, where the ErbB2 receptor kinases is expressed in otherwise wild type mice, and you can see that they fairly rapidly develop multi focal tumors and by about 170 days all mice have developed tumors.
So we then added one additional mutation, which is to incorporate into those mice a floxed allele of the ShcA scaffolding proteins, so their homozygous for conditional allele, such that the action of Cre recombinase would inactivate the ShcA gene.
So you can see quite a remarkable result, that those mice as well as their virgin mice, are entirely protected by the development of mammary carcinomas.
So that at least is consistent with the notion that the ShcA docking process is largely take place important downstream of ErbB2 and is being exploited in this system by ErbB2 to drive the rapid development of mammary genes.
So let me stop there.
I've argued that modular interaction domains provide a rather general mechanism to organize cell regulatory pathways by showing you a few examples, and then I've argued that aberrant protein interactions promote disease and I've given you a couple examples, one that has to do with bacteria pathogenesis, and other with the development of malignant translation.
Stop there, in fact my colleagues, Alec Cheng, Claire Chazaud and Janet Rossant, did the work on Grb2 that I initially alluded to, then the work on Nck was done primarily by Nina Jones and Ivan Blasutig and Jim Fawcett, the work on the kidney, a collaboration with my colleague Sue Quaggin and the work on the node system with John Georgiou and John Roder, and then the bacterial work was a collaboration with Sam Gruenheid and Brett Finlay, and again the work on ShcA was work done by student from the lab, Rod Hardy, together with Bill Muller, his colleague.
Stop there.
Thank you for your long-suffering attention, and I'd be happy to take questions.
[ Applause ] >> All right, we'll grab some questions.
Brian?
>> In -- I don't know if this is possible, but in those mice [inaudible] paralysis on one type of a mice?
Would the Nck deficien5t mice be able to recover?
>> So the question is if we were to consult these mice by inducing a stroke of tail, let's say on one side, how would that affect tissue repair, which we haven't done.
It's a very interesting question.
I should say, not quite in answer to your question, but we do know that Nck has additional functions in the nervous system, I didn't go into this in detail.
We were sort of surprised with this very specific thing, but it turns out in fact that in those mice, there's still some residual Nck so they're not completely null for Nck2 and if we reduce the level further we start to see some additional phenotypes.
But interestingly -- so I told you that Nck1 null mice are viable, and I didn't say when one usually says something we say they're viable and happy and I didn't say they were happy.
Because they do get spontaneous seizures, and they show the sort of scurrying depolarization, which again was stuff to look at, but it's sort of rather like a sort of thing you'd see in sort of migraines.
But there's some effect on synaptic function in that, and [inaudible] play into these disease modes.
but that's one of the theories of model we have is this sort of seizure like model.
So Nck1 itself does have a function [inaudible].
>> David?
>> In close to the end of the experiment with ErbB2 ShcA and Cre recombinase, an alternate explanation might just be knockout ShcA and kill the cell or something.
That has nothing to do with ErbB2.
Are there controls like that?
>> Yeah we can certainly show -- yeah, sorry, the question is how do we know that we're not just killing the cells by deleting ShcA [inaudible].
So if we -- and you say experiment so leave out the ErbB2 just sort of select the lead ShcA -- it gives us the same phenotype as ErbB2, actually, which is that the mammary -- the progression of the mammary epithelium is delayed, but then it's normal.
So you don't absolutely need ShcA the mammary into develop.
So another -- so I showed you as a sort of artistic device these sort of virgin mice [inaudible].
Once they start to have offspring, a few of those start to develop tumors, [inaudible], and those tumors lack ShcA, so they sort of found a way of getting around ShcA, and they seem to be expressing [inaudible], which is an interesting issue.
Yeah?
>> And do you know if the ErbB2 positive ShcA negative cells die acidosis, or did they -- >> They seem -- in the ErbB2 model, they seem to survive, and we have other models -- there's also a big study that [inaudible] and we have a whole Elitic series of ShcA, and there is one ShcA site that seems to couple specifically to survival in the [inaudible] setting, so [inaudible] driven breast cancer seems to be more dependent on ShcA for their survival than do ErbB2.
ErbB2 you get a little bit of [inaudible].
>> Do you ever see an adaptor like Nck mobilized with a positive receptor.
Or are these interactions too weak and transient?
>> [Inaudible] >> Yeah. I was sort of -- so I mean, it's an excellent question, actually because you know, it's the kind of thing one would like to do now sort of better by imaging.
We didn't really have a set of dynamics nearly as well as we should.
Nephrin is perhaps a bit of an unfair example because it has sort of three really good Nck binding sites.
Nck has three [inaudible].
>> [Inaudible] >> Yeah, but here we think -- this experiment, we can see, so here you've got nephrin in green, sort of Nck in blue and we've got this sort of backed in tail, and we see the same sort of thing with Tir.
So here because you've got these very strong multiple binding sites, you can see this kind of thing, but obviously now we're trying to use things like photoactivation of the adaptors and so forth just so it'll look more [inaudible].
And the operants of these things are actually quite high, so you know, but things are obviously more complicated.
[Inaudible] for example, has a very protein rich, long protein rich sequence, so we have some data that suggests that multiple Ncks combined with the same and WASP and those different Ncks could then either bind to multiple sites on the same receptor actually sort of cross linked receptor, so clearly, what's really going on is much more complicated.
>> Thanks.
>> With Nck and the activation, how much of it is a spatial control where you're bringing things to a membrane using Nck as the adaptor versus a real activation where the SH2 domain tells the SH3 domain to do its work?
>> No, that's sort of a -- you mean sort of feeds on string in a sense or?
>> Well, the bottom line is in theory you should be always interacting with WASP.
>> Right, so interestingly in this system we're seeing that the Nck -- so in the nephrin Nck and WASP system, we see, when we cross link different -- an inducible interaction of Nck and WASP, the reviews of the paper all say, okay, that's not -- show them.
So we don't understand why as yet, but it's worth thinking -- you know, again, I sort of portray that as a very simple [inaudible], That's far from the case.
So if you think of it crack, it has an SH2, and then 2, SH3 domain So out.
So we showed some years ago, as did [inaudible], that so we showed -- that when crack -- see, crack divides into this issue leans to scaffolds in terms of kinase, becomes phosphorylated.
And then that phosphorylation site interacts with an internal SH2 domain, which then results in its falling off, being displaced from the receptor.
But interestingly, a recent structure cover this, showing that in the sort of naïve state, the SH2 domain of crack interacts with its own SH3 domain in a fashion that protects them both from binding to external ligands, a bit perhaps like the famous [inaudible].
So then you can imagine, so the adaptors are like this, something gets phosphorylated, this SH2 domain comes off, binds to a phosphotyrosine site, the SH3 domain can now bind to a target, but then the adaptor that's phosphorylated has an issue when I it comes back, binds to a tyrosine site so it falls off.
So that's all that we know about crack.
Nck, the ultimate [inaudible] interested in this issue, but all that we know is when the nephrin Nck, binding there's some nice data from Mike Rossin, suggesting that N-WASP must be at least dyamarized to the [inaudible] sort of model suggesting that [inaudible] imaging that Nck [inaudible] N-WASP is contributing to the N-WASP polymerization of N-WASP3.
>> Is there any indication that the SH3s and Nck have somewhat distinct proline rich motifs specificities?
>> Yes. They do have distinct specificities, yes.
[Inaudible].
When you've taken all of this, put them back, it seems like each SH3 domain has some activity, but [inaudible].
>> More questions?
If not, there's a reception right out here and stick around.
[ Applause ]
About the speaker
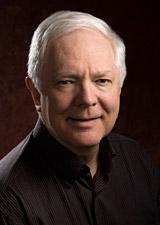
Anthony Pawson
Distinguished Investigator, Samuel Lunenfeld Research Institute of Mount Sinai Hospital & Department of Medical Genetics and Microbiology at the University of Toronto
Tony Pawson has pursued the mechanisms by which cells in the body communicate, and the aberrations in the process that result in diseases such as cancer. In particular, he has focused on the means by which growth factor receptors at the cell surface are linked to their intracellular targets. This led to the identification of the Src homology 2 (SH2) domain, the prototypic protein interaction module that exerts its effects through the selective recognition of phosphotyrosine sites on activated receptors. It is now apparent that modular protein-protein interactions control many aspects of intracellular signaling pathways, and provide a general architecture for organizing cell regulatory systems.
Tony Pawson obtained his Ph.D. at the Imperial Cancer Research Fund (now Cancer Research UK), working on retroviral gene expression. He undertook postdoctoral work at the University of California at Berkeley, where he identified a variety of retroviral oncogene products, and provided early evidence for the role of tyrosine phosphorylation in malignant transformation. He moved to the University of British Columbia, Vancouver as a Assistant Professor in 1981, and then to the Samuel Lunenfeld Research Institute of Mt. Sinai Hospital, University of Toronto in 1985, where he was Director of Research (2000-2005). Over the last 20 years he has explored the mechanisms through which signaling networks within the cell are constructed, building on his discovery of the SH2 domain, and the ways in which such networks are utilized to develop functional tissues, as well as the defects in molecular interactions that promote human disease.
Dr. Pawson is a University Professor of the University of Toronto and a Distinguished Scientist of the Canadian Institutes for Health Research. He has received many awards, including the Gairdner Foundation International Award, the AACR/Pezcoller International Award for Cancer Research, the Heineken Prize for Biochemistry and Biophysics, the Louisa Gross Horwitz Prize, the Wolf Prize in Medicine, and the Royal Medal from the Royal Society of London. He is a Fellow of the Royal Societies of London and Canada, a Foreign Associate of the National Academy of Sciences (US), and an Associate Member of EMBO. He was recently appointed by Queen Elizabeth II to the Order of Companions of Honour.