
Genetic Control of Programmed Cell Death in C. Elegans
Transcript
>> -- X from regulating ced-3.
Ced-4 promotes the activity of ced-3.
And ced-9 protects by preventing ced-4 from promoting the killing activity of ced-3.
So this raises the question, well, what then controls ced-9, okay?
And I think I'll only go through this quickly because of the hour, but the answer is Barbara Conradt [laughter] found another killer gene, egl-1.
In fact, this is the same gene I've told you about before, but the mutation I told you about before was a special mutation in that gene that causes that egg-laying neuron to survive.
If you turn the gene off, you see exactly what you see if you turn off ced-3 or ced-4.
Egl-1 like ced-3 and ced-4 is a killer gene but unlike ced-3 and ced-4, it acts upstream of, not downstream of ced-9.
So egl-1 kills by preventing ced-9 from preventing ced-4 from activating ced-3.
[Laughter] Barbara cloned egl-1 and it turned out to also have human counterparts.
These human counterparts are members of the Bcl-2 superfamily, but these proteins are not protectors, they're killers and the family members can interact together, protein to protein.
And in fact, overall, this core molecular genetic pathway looks like this with egl-1 to ced-9 to ced-4 to ced-3 interacting physically, this protein with that, this with this, this with this.
And furthermore, as predicted from this pathway, the counterparts in mammals including us act in essentially the same way, okay.
So these are the basic findings that have come from these studies.
And if you think about it, just stepping back, it's easy to remember the outline of what I've told you.
Four basic steps in programmed cell death, okay?
One, who's going to live, who's going to die.
Two, make the ones that are going to die dead.
Three, you've got to get rid of the body.
And four, you want to store all of whatever's left over, and that's it.
That's the essence of programmed cell death.
Now, the details are a bit more, okay?
The pathway is a little more complicated.
This pathway is gone.
It's a little bit out of date.
I'll update some of these tomorrow and give some more ideas.
But basically, the essence of what I've described is the soul of the killing part of programmed cell death and what I've told you about is how that was found.
Now, let's think about that in the context of disease, remembering what I told you at first.
Think about these genes, not the worm genes, but the human counterparts.
Nobody wants to cure worm diseases that I know of.
[Laughter] Diseases caused by worms, yes, but not diseases of worms.
What about therapeutic targets?
Well, if you take a killer like a caspase and inhibit it, you will prevent caspase action and you can prevent programmed cell death.
So diseases in which there is too much programmed cell death, diseases like certain neurodegenerative disorders, heart attacks, heart failure, liver diseases, and so on, these are diseases where caspase inhibition offers a promise.
And there is currently in phase three at a major pharmaceutical company a drug directed against caspases with particularly liver disease as an indication, liver disease in which there is too much programmed or apoptotic cell death.
On the other hand for diseases in which there's too little cell death, you can inhibit a protector like a member of the ced-9/bcl-2 family.
And if you could prevent protection, for example, in cancer cells and cause those cancer cells to die, that could have a major therapeutic benefit.
And there is a phase 1/2 trial at another major pharmaceutical company underway with exactly this approach with cancer as an indication.
Okay, so with those comments, can I take a couple of more minutes because I want to turn to something that's very different and I want to put these comments in a broader context?
The work that I've talked about here involved absolutely basic research.
When I began, neither the generality nor the application of the efforts was it all there.
C. elegans was an obscure organism.
In fact, I've been advised that it was a professional dead-end to work on it.
Genetic studies are often highly abstract and very formalistic.
Nonetheless, the studies that I've described established mechanisms that appear to be universal among animals and our findings may well help lead to treatments for a broad variety of human diseases.
And I think there are a number of messages here.
The first and most fundamental is basic research, truly basic research.
Research that is discovery-oriented, research where you don't know what you're going to find is crucial.
It will lead to intellectually stimulating things and in findings, and that's very important.
And it also will lead to major insights of broad practical import.
And we have example after example of such findings for all kinds of fields in biomedicine and in science, generally.
Basic research is the driver of biomedical knowledge.
The second thing to keep in mind is who fund basic research.
Essentially, you've got governments, foundations and charities, state, federal, and so on, but it can't be supported in the commercial sector.
You can't define basic research as I've described it as is crucially important in making business plan out of it.
You have to have support that is directed to basic research.
So as one thinks about problems and problems that are in need of research and diseases, diseases that we really care about, we need knowledge of disease but we also need knowledge that we don't even know where to begin to ask about.
No one would have started with the kind of work I described focused on any particular disease.
And again, I picked from this because I was involved many, many examples.
Okay, so let me finish.
I'm going to skip some of my more political slides because things have changed a little bit in the last day.
[ Laughter & Applause ] And simply talk for a minute about one of the findings that I think has been so striking for modern biology, and that's a finding that I referred to as the principle of biological universality.
I mean the fact that you can study a worm or a fly or a yeast and learn about how to treat human diseases is pretty remarkable.
And I think those of us who have been involved in this field for some years had been pretty excited about these findings, but one always has to be careful about pride because sometimes where you think you are threading for the first time are areas that have been well thread before-- well trod before, I guess is the right word, got to be.
And so, just thinking about the findings I described today, I want to show you the cover of Punch from 1882 where it's clear that the artist already knew what it took many of us some years to find out, Man is but a Worm.
[Laughter] And with that, and thank you to the people who are in and have been in my lab and I will note the red hair, not as my student.
[Laughter] George, I had the pleasure of hosting George for a sabbatical visit some years ago.
These are the people that I thank and I thank you as well.
[ Applause ] [ Inaudible Conversations ]
- >> Volker Vogt
Racker lectures and more of the lectures given to us by Dr. Robert Horvitz with MIT who had been in conference last week at MIT.
I think those of you here last night heard his full CV, I won't give you all that again, but I'll give you a few comments since I made a few a few personal comments.
Bottom line, we've been in a few of the same places.
We had some similar and some different experiences with those places.
So Bob mentioned earlier in science [inaudible], I think, he started out, as you heard last night, he's an undergraduate majoring in Economics, he got that, and then he jumped in Harvard into the Watson-Gilbert, Gregor [phonetic] and some other people gave him a lot, this huge-- created as an interesting but difficult place as well.
He worked as a graduate student on transcription-- bacterial transcription which is what I did as well and which I have been here for carefully for the last four years as well, but-- in his theory seminar.
And after that, I will be interested rather [inaudible] when he found at the MRC afterwards another fascinating and creative place particularly at that time.
And again, I worked on bacterial transcription, but he chose one of the directions that [inaudible] taking at the time [inaudible] framed by Crassus Craig [phonetic] and Sydney Brenner, this sort conversational energy dominated [inaudible], sometimes the [inaudible] dominated the [inaudible] couldn't escape, Sydney Brenner wouldn't want to talk to you.
[Laughter] At the time-- and a lot of it is changing, finding new direction, trying to find development in eukaryotic systems to work in.
There could have been loss of general direction, actually.
Of course, the major work is the nematode, the development of genetic system which Sydney Brenner developed.
There were other things going on at the time which I don't think [inaudible] could sit upon and there's a notion of mapping the entire nervous system wiring [inaudible] continues at the time occupied by [inaudible].
There were other organisms being explored, but the major ones was the nematode.
And it was clear that Bob tells exactly the right path that he's involved his experiment with mapping out all the cell fates last night.
And then he took from that the central ability to do classical genetics and study development as well.
You should look at, it as classical genetics.
We need to generalize basic map and [inaudible] all sorts of [inaudible] which led among other things to discover the pathways of programmed cell death that won him the Nobel Prize in 2002, probably many other directions as well.
So, it's a great pleasure to see Bob here and welcome here-- welcome him here.
He's going to talk about today genetic control of programmed cell death in C. elegans [inaudible] continue the lecture, Bob.
[ Applause ] [ Pause ]
- >> Robert Horvitz
Does this work okay?
You can hear me with that?
Just kidding.
Of course, you can [inaudible].
[Laughter] >> Don't put it too close to your mouth >> What? [Inaudible Remark] Too close.
No, that-- I don't think that-- I think it'll do.
Jeff, where did you go, Jeff?
Thank you.
[Laughter] One thing that Jeff didn't say is that during my newly, early informative years, Jeff was my mentor.
He was in the room, the lab next door to mine, and I think I drew upon his expertise and his advice constantly, relentlessly and he had a very, very major role in helping to define me as a scientist, both in terms of keeping me enthusiastic when things didn't work and keeping me excited when things looked promising.
He really is responsible for defining me.
I was in the first successful experiment, you know, the one that really made a difference in terms of my background on defining the goal of that experiment from the get-go.
So I just want to say a special thank you to Jeff for all of that some years back.
And more generally, I want to thank everybody who has posted me over these two days.
This has been a fantastic visit.
I know it's kind been kind of whorking.
So all of my conversations have been cut short, so I'm sorry about that, but it's been very exciting.
I have really gotten to see talk with number of people, some old, some new, and I've learned a lot and I've been excited.
And one mark of a successful visit from me is I think some of the things that I've learned had actually impacted [inaudible], talked to him a lot about it, so that it makes it very much special, special fun.
Okay, so to the topic at hand here.
I was trying to consider what to do with the second of these two lectures, whether I should address a topic that is completely different from what I talked about last night, or really extend the topic that I chose for the general comments from the general audience last night.
For the continuation, a hefty number of conversations this morning, I'm wondering if I think I should have selected one of the other topics that [inaudible] with another set of people, but what I've had together is the continuation.
And so, that's what you're going to hear.
And I thought since I know not everybody was there last night, I will spend at least a couple of minutes at the beginning of just reviewing the take-home and then turn to some of the newer work and discuss that for this hour.
So in brief, this is the C. elegans cell lineage.
Many describes the patterns of cell division starting with a simple fertilized egg, generally and ultimately, the 959 cells that are found in the adult animal.
Its lineage was worked out the mostly by John Sulston at the same lab Jeff referring to, the MRC, Medical Research Council LMB Laboratory or Laboratory of Molecular Biology in Cambridge, England.
And in addition to the 959 cells that were generated in this lineage and found in the adult, there are 131 others that are generated not from the adult and the reason is that these cells systematically reproducably die.
They undergo the process of programmed cell death.
So we embarked upon genetic studies.
I discussed the beginning of these studies yesterday, looking for mutants that were in one way or another, abnormal in this process of programmed cell death.
And basically, these genetic studies defined a four step genetic pathway that corresponded nicely with the biology.
The first step was the decision that basically every cell has to make "should I live or should I die" by programmed cell death.
For those cells that decide to die, they must quite literally execute that decision and take a happy healthy cell and make it dead.
The dead cells are going to be engulfed by the process of phagocytosis, of removing the cell corpses and then there is a degradation of the macromolecular debris of the cell corpse.
And what I described yesterday was our earlier studies that led to the definition of a molecular genetic pathway in which we had found that ced-3 which encodes protease what later became to form as the caspase family is responsible for killing and then the set of four genes that encode proteins that interact physically and in controlled cell death in the caspade by which egl-1, the killer gene, kills by preventing ced-9, a protector gene, from preventing ced-4, a killer gene, from activating ced-3, a killer gene.
And this was the pathway in essence that I finished with yesterday and explained that, in fact, all of these genes have human counterparts and the human counterparts probably act in analogous ways.
In fact, best known of these is probably bcl-2 in humans which is involved and was discovered in studies of human cancer and causes cancer because what ced-9, it is a and an anti-apoptotic gene [phonetic].
And when cells are protected from death, and it should die, that can promote basically a cancerous cell division, okay?
So that's essentially what I said in our-- yesterday.
So now, what I want to do is to update the story a bit, and then I'm going to focus on not on that core killing step, but rather on really what was a question asked at the end of the lecture yesterday which is how is this core pathway breaking it and [inaudible] a little about that.
So first, a little bit of update in terms of the core part.
What I've said here is that this is the core pathway for programmed cell death, but it turns out that some cell deaths occur independently of these.
And in fact, it turns out that not only ced-3, the caspase, but two other functional caspase genes are present in the C. elegans genome.
And if you eliminate the ced-3 caspase or, in fact, eliminate, we've made a deletion mutants, systematically, all of the caspase genes, you, nonetheless, still get a little bit of programmed cell death.
So it is clear that cell death can occur in the absence of the core cell death pathway and that it can occur independently of any recognizable caspase gene.
So there is some other mechanism or set of mechanisms that exist that are clearly present which play a biological role and we do not, at this point, know what they are.
And part of the message I want to leave you with is despite what I'm telling you yesterday and today, there is a lot that we-- that remains unknown about how cell death occurs and about how cell death is regulated.
So the first message is there is caspases that can lead death, I won't go through the details of the greater.
That's just a take-home message to keep in mind.
The second thing I want to talk about is the gene ced-4.
Now, yesterday, I described ced-4 as a killer gene and that was the message I gave you in the summary just now.
What I want to tell you, also, that the reasons to suspect that ced-4 may be bifunctional.
It may not only have pro-apoptotic but also anti-apoptotic killing function.
There are a number of reasons to go-- to suspect that.
I won't go into all of them.
I'll tell you one set of experiments from some years ago and then I'll tell you an update on some new experiments we're doing now that support the hypothesis that ced-4 has both pro-apoptotic and anti-apoptotic activities.
Okay, so some years ago Shai Shaham who's a graduate student in the lab, and Shai is now in the faculty of Rockefeller, he discovered that ced-4 has two alternatives splice forms, a short form and a long form.
And he actually found this from early comparative genetics.
He just was looking at different species and he found, as we now know is very common, that coding regions are conserved, non-coding regions are less conserved, and he discovered that there was a conserved non-coding region that happened to be 72 bases long, it could divide, 72 divided by 3, was 24, and he postulated, "Oh, maybe these 24 extra"-- oh, and the 72 basically constituted an open reading frame that would be consistent with the 24.
When he looked at the sequences, it looked like there might be a splice site that would be an alternative site.
He tested that and, in fact, he had found a minor ced-4 message species that was 72 bases long.
So we have ced-4 short and ced-4 long.
And then to functionally study these, he used them as transgenes, put them into worms that lacked ced-4 function 'cause we had no mutations in the gene and the assay for killing activities.
And he found that ced-4 short had very potent killing activity in specific assay which I won't go through.
And ced-4 long did not have killing activity no matter what he seemed to do.
And then, just through the course of experiments, he discovered that actually ced-4 long not only didn't have killing activity but actually it had protective activity.
And so, from that, in the series of experiments, he postulated that ced-4 short and ced-4 long have antagonistic activities with ced-4 short, the major form, and the primary functional form from the genetic studies as killing activity and ced-4 long has, at least in these transgene experiments, protective activity.
And then he also obtained in vivo genetic data that if you really tweak the system and balance on a knife edge between life and death, you can show that by reducing ced-4 function, you can actually reduce protective activity in the appropriate genetic background.
So that says that in vivo, it looked like there is some function as well.
Those were experiments done, again, some years ago and they were kind of put on the shelf to think about until very recently.
And what's happened recently is a graduate student, Brendan Galvin, has made some observations that reinforced this hypothesis about antagonistic functions for ced-4.
And specifically, what he was doing was looking for new genes that led-- like ced-9, the Bcl-2 counterparts, are protective.
And the way he did this was to basically use the approach-- [inaudible] describe [inaudible]-- use the basic approach of suppressor genetics, again, hoisting the system in a way that if you have a little bit of protective function, you ought to be able to detect it, and using an assay that would be particularly sensitive.
And let me tell you first about the assay then about the experiment and then about what Brendan found, okay?
So the assay was one that was worked out by postdoc Scott Cameron.
Scott is now in the faculty at UT Southwestern in Dallas.
And what he was studying was programmed cell death in the ventral nervous system of the animal and there, in particular, are six neurons that are generated along here.
This is the ventral side of the animal, dorsal up here, ventral down here, and this is the front, this is the back.
There are six neurons that are generated here from a set of six lineages.
We actually call them sub-lineages that are identical.
So in each case, this neuron is the posterior daughter of an anterior daughter of an anterior daughter of a cell that looks very much like the other six here and it has very similar properties.
So they're developmental homologues.
And it turned out that these six homologous neurons are also homologous by decent with another set of cells, with another set of seven cells in which the corresponding cells by lineage die.
They undergo programmed cell death.
And what we knew already was that if you prevent these deaths by, for example, a ced-3 caspase loss-of-function mutation, these other cells survive and they take on attributes of these neurons called VC neurons, okay?
So these, we call them undead cells, become VC neurons.
And on this diagram, I labeled the neurons into green because we use a reporter that we came about-- came at from other studies, some related studies, that it is expressed in these cells and we [inaudible] was important.
So the upshot of this is that if you take an animal that is mutated in that course of cell death pathway and is, in fact, just a slight loss-of-function of that pathway, for example, what he used here was a weak-- a ced-4 mutation that did not totally inactivate ced-4.
There was a little ced-4 activity left.
So instead of making the particular assay to use, there would be five extra cells reproducibly.
If you inactivated the cell death pathway, he got four of the five cells in this animal that almost, but not quite, was ced-4 function.
And then he mutagenized looking for suppressors of ced-4, okay?
And the way to look for suppressors of ced-4 is to go back to the wild type pattern i.e. have more cell deaths, and what he was looking for there is genes that would mutate the loss-of-function and that loss-of-function would cause more cell death.
Therefore, loss-of-function would be in a gene that was a protector, okay?
That was the rationale.
Okay, and he got a number of things out of that and I'm going to tell you only about one mutation.
The mutation was in the gene called spk-1, and I'll tell you why they named it in a moment.
And he characterized the gene genetically.
He isolated a deletion allele of this gene spk-1 and what he showed, as I described, is that spk-1 loss-of-function, internal spk-1 mutations, in fact, suppressed the partial loss-of-function of ced-4.
So here are data.
So you have little data in two days.
Here are data that show essentially what the basis of the screen was.
Here's the ced-4 mutation.
If you're completely blocking cell death, you would be here.
This was almost but not quite it could be blocked in cell death.
And with the spk-1 mutation, here's the deletion allele.
You get a lot less-- you get a lot less blockage.
You get fewer [inaudible].
You get more cell death, okay?
And these are numbers that essentially say the same thing.
More generally, what we found is that these spk-1 mutations would suppress weak ced-4 mutations, but they wouldn't suppress mutations that were total loss-of-function for ced-4.
So you needed some ced-4 function in order to get the suppression.
So what is spk-1?
Well, it encodes a protein a member of a conserved family called SR protein kinase, hence spk.
And this gene, by just homology, lightly acts on regulating splicing, okay?
That's the hypothesis from just the homology.
And if you think about this, and again without going through all the data 'cause this isn't the main focus of what I want to talk about now, the model is actually very simple.
The spk-1 controls the phosphorylation of one of these SR proteins and that basically, the state of that SR protein or set of SR proteins controls the use of the splice site that specifies what would you get, the long or the short splice alternative and that, in turn, regulates the amount of pro-apoptotic and anti-apoptotic function that you get just according to the very simple model.
And Brendan's done some experiments, but they're in the very early days where the data looked like they could be consistent, but I wanted to say that not everything is reproducible and I'm not going to swear that this is going to hold up.
But if you look here, for example, here is the short transcript and here is the long transcript.
And in the spk-1 mutation, you see an absence of the long, that's an absence of protection, so that's consistent.
And that's, at least, the working model.
There also is an allele of ced-4 that from earlier data we think is involved in controlling the splice effects on ced-4 to ced-4 allele and these spk-1 mutations have no effect on this weak mutation which is involved in controlling the difference between splicing because it affects the splice site, suggests selection itself.
And I'm, again, not going to go into the details of this.
But the data are consistent, and this is really all I wanted to say now, with the model that first ced-4 has alternative splice forms and it not only is a killer but the alternative splice form can be protective and that this is regulated by the-- at least, the spk-1 gene and even the way that is, in fact, very simple to think about as part of the model.
Okay, so let's sort of update number two.
And now, what I really want to turn to is this question that was raised at the end of my talk.
I gave you this core pathway beginning with egl-1 and the obvious question then is what regulates egl-1.
If egl-1 is active, a cell dies, if egl-1 is inactive, a cell is going to live, what regulates egl-1?
And the answer to that has come out of a series of interrelated experiments.
So again, here's the core and what's regulating this.
And it began with studies of this gene of-- studies of egl-1 and the genetic analysis of egl-1, and I alluded to this yesterday.
There are two classes of egl-1 mutations.
Loss-of-function mutations, no programmed cell death and that's the statement, egl-1 is a killer.
But we've also had and, in fact, discovered egl-1 in different way.
Egl-1 gain-of-function mutation.
These are confirmed a dominant phenotype and there are, in essence, too much egl-1 activity of a very specific sort, it turns out.
They made to this egl, egl-1 in defective phenotype, because they caused particular set of neurons that I described yesterday to undergo programmed cell death, okay?
And the question then is why is that?
Well, we had seven independently isolated gain-of-function mutations of egl-1.
And quite strikingly, all of them mapped quite a distance by C. elegan standards from the egl-1 open reading frame.
They were 5.6 KB away and they were all downstream and localized to a small region here, and I'll come back in a moment to the precise sequence changes that they involved.
But first, I want to say a little bit more about biology and, specifically, the biology of HSN neurons.
Remember what I told you yesterday, if you were there, about what HSN neurons do.
They control egg-laying, okay.
And in fact, the name, HSN means hermaphrodite-specific neuron.
C. elegant has two sexes, one is male, one is not, okay?
The hermaphrodite lays eggs, the male does not.
A hermaphrodite has egg-laying neurons, HSNs, the male does not.
And the reason the male doesn't have HSN neurons is that they undergo-- in males, they generate, but they undergo programmed cell death.
This is a sexually dimorphic programmed cell death.
So with that in mind, we postulated that maybe what's going on in this egl-1 gain-of-function mutants is a sexual transformation in the identity of the HSN neurons, and I wouldn't be postulating that if, in fact, that didn't turn out to be the case.
[Laughter] What is going on now, empirically, is in the egl-1-gain-of-function mutants, the HSNs die, the hermaphrodites, and the reason [inaudible] they are expressing what is normally a male-specific cell fate.
Okay, now that says that something about sex determination for the HSN neurons must be perturbed.
Well, what do we know about sex determination in C. elegans?
And the answer is a lot.
There has been a whole history of analysis of the sex determination pathway pioneered and pursued by Jonathan Hodgkin followed by probably a variety of other laboratories ending up in a pathway that looks like this starting with an assessment of the extra chromosome ratio and ending in a gene called tra-1 which is the same thing for transcription factor which is determining sexual identity.
When this gene is active, you get a female fate.
When it's inactive, you get a male fate.
In fact, the way you get a hermaphrodite is you transiently turn off tra-1 in a female and make some sperm, okay?
So this is the key sex determination gene and it is a transcription factor.
Why have I told you this?
Because this says that, in fact, one aspect of this sexual metamorphism is HSN survival.
These seven egl-1 gain-of-function mutations fall in a very small region that matches, by sequence, the consensus binding site for tra-1, a binding site that-- [ Noise ] Okay, I think everyone just save that for the moment.
Okay, so that says that you have this-- and if you look, the seven mutations define only three distinct single base pair of changes.
The biochemistry very nicely supports this.
All three of these changes show that these gel shifts experiments that there is greatly reduced affinity for this region of the egl-1 downstream sequence by the tra-1 promoter-- by the tra-1 protein.
And overall, this leads us to a very simple and, in fact, over simplistic model for what's going on mainly in wild type hermaphrodite, sex determination, in fact, where you put tra-1 on.
Tra-1 binds to this site that is a cis-acting regulatory site which causes repression of the egl-1 locus.
If egl-1 gives off, egl-1 is a killer, if egl-1 is off, HSNs live.
In males, follow the one [inaudible] sex determination pathway, egl-1 is on and the HSNs die.
And then egl-1 gain-of-function mutation, tra-1 is on, but it can't bind, so egl-1 is still on in these cells, in that case, will die.
Okay, so that's simple one.
First reason it can't possibly be all there is.
Two, it is-- if that's all there is to it in egl-1 gain-of-function mutation.
Or in fact, for that matter, in a male, every cell would die.
And the answer is, as we know about transcriptional regulation in general, one transcription factor isn't enough to specify what goes on.
This transcription factor works in combination with other transcription factors.
And in fact, these other transcription factors have been identified by our comrade who is a postdoc in my lab worked out the story I just told you about egl-1 regulation and tra-1 and then in [inaudible] to identify some of the interacting transcription factors.
And the upshot is these transcription factors work together.
And basically, what they're doing is they're saying, "I am HSN neuron," define himself identical-- identity the way transcription factors do very generally in development of biology, "and I am male or hermaphrodite." And the two together determine the transcription of the egl-1 gene which, in turn, determines whether or not HSNs live or die.
Now, one thing that's interesting about this is that these genes, one, in terms of human counterparts do have counterparts.
Tra-1 is a member of the Gli family of transcription factors.
And the tra-1 homolog would be, one, is over expressed in most, if not all, basal cell carcinomas.
These genes, the [inaudible]-1 gene translocations involving the counterpart that's been associated with acute promyelocytic leukemia and also with apoptosis.
So the postulate is, first, that these genes have counterparts that are involved in controlling cell death.
And secondly, that if something goes wrong, they can lead to cancer.
Okay, now in a second study, different set of neurons, looking again, at the cell-type specific control of programmed cell death.
The picture that emerged is very simple.
This time, four genes regulate egl-1 transcription, four different transcription factors.
Again, the homologues have been implicated in apoptosis in mammals.
And again, the homologues have been implicated in human cancer, this time in ALL, acute lymphoblastic leukemia.
Okay, so we have two examples now of cell-type specific regulation of programmed cell death and the generalization is very striking.
It's done by transcription factors which interact to work on a cell by cell basis to regulate the transcription of the gene egl-1 and counterparts back in apoptosis and in cancer.
So given these findings, we decided to look at more cases.
So that for example, we look back at these VC neurons or the ventral cord I told you about before.
And remember, the cells and the assay and all of that.
And the two graduate students, Brendan Galvin whom I mentioned and Peter Reddien who is now a faculty at the Whitehead Institute [inaudible].
They used this same assay to seek mutants that were defective in these programmed cell deaths, okay, to ask whether they can get a handle again on the cell-type specific regulation of programmed cell death.
And what they did is they started, again, with a poised allele of the cell death pathway.
But this time, instead of ced-4 allele that almost, but not totally reduced killing, they took a ced-3 allele that had very little effect on killing.
We have now-- we have over a hundred alleles of ced-3.
And you might ask how we have ced-3 alleles that have no effect, and that's another story.
But we have a lot of alleles and we could easily, you know, balance this pathway exactly the point we wanted.
And they took this allele that has just a little bit of effect on cell death and they looked for mutations that then increased the ced phenotype, the opposite screen of the one I just described, and answers a defect in cell death.
They found a number of mutations, and again I'm only going to tell you about one of those now.
This mutation which we call n4045, which means it was the 4,045th mutation characterized in the lab, it turned out to be an allele of egl-1, okay?
And it blocks the cell deaths of the VC neurons.
It is an enhancer of the weak ced-3.
It has a pretty good, but not total effect on the cell death of the VC neurons.
Looking at other cell deaths-- I mean, here's one example with another region of the animal.
It doesn't do anything, okay?
It is specific for the VC neurons with respect to a large number, I can't say all, but at least many, many cell deaths in the animal, okay?
Now, in an independent screen in the lab done by another graduate student, Dave Harris, Dave was looking for specifically new alleles of egl-1 dping what we call a non-complementation screen.
He was looking for mutations that fail to compliment egl-1 mutations for the defect in VC neuron life-death decisions, okay?
So it's just a different screen to, again, try to get handle on these cells.
And he came up, in this screen, with one mutation in a new egl-1 allele, n4629.
And the phenotype of n4629 was spot on with the phenotype of the other mutation that I told you about.
And the reason turned out to be they have the same base change.
They are identical mutations and they are in an evolutionarily conserved sequence.
Here, you see two other [inaudible] species.
And they are in a region that is, again, downstream of the egl-1 coding region, okay?
This time, 4.6 KB, not quite as far as the region I told you about before.
And from this study and also we identify a leash of mutants leading this locus which allowed us to put a sign on the interaction of this locus, 309 base pair deletion, we could conclude that at this site, there must be an activator that is binding that activates transcription of the egl-1 gene.
And so, the model very simply is a VC specific activator binds.
Here's the site, a little bit further that I told you about before, activates egl-1 and that causes cells to die.
And if that site is inactive, egl-1 is not transcribed and cells live.
So that's very simple model, second cis-regulatory site, this time, activation.
And what Brandon has done is to now use one hybrid screens looking for proteins that act the site.
And I won't go through on what hybrid is.
And it's done, but the answer, for example, is you get things that turn up and we postulate that at least one of the three proteins that has come up in this screen may be functionally involved as a transacting activator that acts at this site.
And the model, again, is very simple and very simplistic.
And hermaphrodites-- in hermaphrodite, HSNs in particular, Tra-1 represses egl-1, which I've told you before, allowing this gene turn-- gets this gene turned off, egl-1 allows HSNs to just survive.
And in the VCs and the cells that normally would die and unknown activator may be more than these proteins that were identified in one hybrid, maybe not.
That unknown activator binds here, activates egl-1 and causes these cells to undergo programmed cell death, okay?
So that's the basic model from these studies.
Okay, so we've done a variety of studies of this sort.
And in some of our ex-postdocs, including Barbara Conradt, I mentioned, and Scott Cameron who I mentioned but has continued with some studies, have done similar things.
And the picture we have right now is what's shown here.
Egl-1 is regulated at a variety of sites, combination of activators and repressors at the level of transcription.
Different cells use different regulatory proteins and different combinations of regulatory proteins.
And what is-- what happens in each case is that either egl-1 is turned on and that cell dies by programmed cell death, or egl-1 is turned off and that cell survives, okay.
Very simple picture and we're beginning to put together a picture of cis-regulatory region that controls this very key first gene in the core pathway for programmed cell death.
Okay, so from these studies, it was looking like there is in fact this great generalization.
But I'm now going to tell you about a study with the same rationale where we found something that's a little bit different.
Okay, so again, we're looking at some type-specific regulation of cell death.
And I mentioned that the HSN neurons involve a sexually dimorphic programmed cell death.
These neurons live as hermaphrodites that control [inaudible] in males.
There is one other sexually dimorphic programmed cell death in the animal.
It involves the CEM neurons.
There are four of them.
And they are found in males and not in hermaphrodites.
They are chemosensory neurons, and it's believed that what they do is smell the presence of hermaphrodites to allow males to attract towards hermaphrodites.
The sexually dimorphism, again, is caused by sexually dimorphic cell death.
These cells are generated in both sexes and they undergo programmed cell death, specifically in hermaphrodites.
So, graduate student, Hillel Schwartz, decided to look for mutants that perturb the CEM life-death decision.
And he did this by using a recorder that was generated by Maureen Barr who has been in Paul Sternberg's lab in Caltech.
And this recorder is expressed in the CEM neurons.
So if you look here, here is a male.
These are the CEM neurons in the head.
Here is the head.
A male tail also expresses this recorder.
The male basically has two brains, one in its head which it uses for most things, one in its tail which he just uses for mating.
[Laughter] There are a lot of neurons back here.
So if you look at the male, in the head, you see these neurons.
And look at the hermaphrodite, and they're not there.
So, very clear distinction.
And the experiment in a very simple way was they use this recorder, mutagenize hermaphrodites and look for hermaphrodites that glow in their heads.
And presumably, some, may be all of those would have CEM neurons that could be a defect in this life-death decision of the CEM neuron.
And proof of concept here is two mutations.
Here is a mutation that we isolated some years ago, it's defected in the sex determination pathway, and considered very, very weak defect in the sex determination pathway.
It's makes an animal but most very much like a hermaphrodite, but it's a little bit male-like.
And it turns out, it has CEM neurons that are particularly sensitive.
Okay, so we knew this screen, we get genes in the sex determination pathway.
Here is the controlled experiment for cell death, ced-3 minus hermaphrodite.
Look at this hermaphrodite, our complete hermaphrodite with the exception of CEM neurons in the head, okay?
So, a very simple screen.
You just look for like green beacons in the head of these animals, and the Hillel did that.
He got 154 independent mutants and spent his career, his graduate career characterizing these 154.
He essentially studied all of them.
I'm going to tell you only about three that resided in one particular chain.
These three are defined-- notice that we do know about before genetically.
They all are located in the same site along the X chromosome, and they cause a dominant, some are dominant, really, phenotype, okay?
So these mutations cause CEMs to survive in hermaphrodites and the phenotype is dominant.
Well, whenever a geneticist encounters a dominant phenotype, that is a flag because most mutations that cause loss of function cause recessive phenotypes.
Most mutations that confer dominant phenotypes are not loss-of-function.
There are exceptions to both of those 'cause that's generally the case.
So as soon as we see something that's dominant, we say, "Maybe it's not loss-of-function." It's easy to test if you have a deletion that exists, even a multigene big deletion or a region of the chromosome, you look at it, it trans to a wild type chromosome and you don't get your phenotype, you know your phenotype isn't loss-of-function, okay?
It's subject to more sophisticated [inaudible].
So with this observation in mind, the Hillel did a standard genetic approach to ask for loss-of-function mutations [inaudible] geneticists in the audience who's looking for cis-dominant suppressors and you found them.
And what he got then was, first, a mutation n4111 that is a loss-of-function mutation in the same gene as these mutations by all of the standard [inaudible] of non-standard genetic studies.
And what was remarkable about this loss-of-function mutation is that the phenotype was opposite of the phenotype of beginning a function mutation.
You turn off this gene, and what happens now is in males, the CEMs undergo programmed cell death, okay.
You have gain-of-functoin, turns out, you turn on the gene.
And in hermaphrodites, the CEMs that should undergo programmed cell death in stead survive.
This is the phenotypes.
So here, you see a gain-of-function hermaphrodite, you know.
There they are the CEMs.
You're going to see a loss-of-function male.
The rest of the recorders express to the tail, CEMs are gone, okay.
Varied between phenotype.
The genes call ceh-30, I'll comeback to that in a moment.
And it turns out to be a cell-type specific anti-apoptotic change.
It works by protecting the CEM neurons against programmed cell death.
When it's on, CEM survive.
That's normally the case in males.
When it's off, CEMs die.
That's normally the case in hermaphrodites.
You make a ceh-30 loss-of-function.
And now CEMs die in males or, let's say, to regain a function, now CEMs survive in hermaphrodites, okay?
Very, very simple picture.
So when Hillel cloned a genes on-- he found that it encodes a homeodomain containing protein of the same in C. elegans homeobox gene number 30 that had been identified by sequence comparisons before, but not part of the genetic analysis.
And this is a member of the Bar family homeodomain, [inaudible] in drosophilas, the bar eye mutant.
It was a very classic mutation and it encodes really the founding member of this Bar family homeodomain proteins.
Now, the loss-of-function mutation he had was a nonsense mutation in the open reading frame they often [inaudible] makes sense loss-of-function.
The gain-of-function mutations, all three of them were located in an intron, okay?
So they're probably regulatory.
Looking at the sequence, it turned out all three were the identical single base pair change.
Furthermore, if you look at this sequence and you have a very good memory, it matches the sequence I showed you before.
It matches that consensus site tra-1 binding sequence.
And in fact, this residue is identical to one of the classes-- three classes of mutations I told you about in the cis-acting egl-1 regulatory region.
It's the same mutation that perturbs the binding of the tra-1 sex determination zinc finger protein, okay?
And what do we know about CEMs?
They're sexually dimorphic.
What controls that?
Tra-1. Who binds here?
Same business, okay?
These mutations, these-- you can see it up here, yeah.
This same mutation perturbs tra-1 binding in the ceh-30 intron as perturbs the corresponding mutation perturbs binding and the egl-1 downstream regulatory site, okay.
So gives us a simple model and some of the models I've drawn before.
In a wild type animal, the sex-- in a wild-type hermaphrodite, the sex determination haploid puts tra-1.
Tra-1 binds to this intron that represses ceh-30, so ceh-30 is off.
Ceh-30 is anti-apoptotic because it is then causing CEMs to survive.
So, you turn off the anti-apoptotic, you don't have protection, CEMs die.
That's hermaphrodite, CEMs die.
In males, conversely, no tra-1, ceh-30 is on, ceh-30 protects, CEM survive, and then you have the gain-of-function and loss-of-function alleles conferring the opposite phenotypes to the two sexes, okay?
Now, what do we know about all of these works?
First, from a variety of studies, we think the ceh-30 is acting self-autonomously in the CEMs.
I won't go through the data.
And secondly, Hillel has asked the question, "What's the pathway?" So if you think about what I've told you so far, you would have and we have a very simple postulate.
We postulated the ceh-30 is a linker between these two cell path-- these two gene pathways of programmed cell death culminating in tra-1 and the egl-1 pathway so that tra-1 regulate ceh-30, this anti-apoptotic gene, which one would then suggest represses egl-1.
Okay, so that was the simple model.
It turns out, that's not the case, okay.
Hillel's data indicate that ceh-30 can act independently of egl-1 and even independently of ced-9, a gene downstream with egl-1, the bcl-2 counterpart.
And I won't go through details of the data.
The upshot here is that if you simply look in a ced-9/egl-1 wild-type background and a ced-9 of, egl-1 off background, you still can get these sexual dimorphism, okay?
So you can do this ceh-30-- and it's ceh-30 mediated.
And you can do this ceh-30 regulation independently of those genes we though among were the heart and soul of the regulation.
And what we think, and this is a model based on a number of things but it's only hypothesis, is that ceh-30 is acting downstream of these two genes to regulate either ced-4 or ced-3 or something downstream of ced-3 in the following way.
We consider ceh-30 to actually poise the sensitivity of the CEM neurons to the programmed cell death pathway.
We know from other evidence that cells that are going to live actually have some killer activity and cells that are going to die actually have some protection activity.
So it's like every cell and some of them are going to live, some of them are going to die, and something sets the set point of that balance.
We think that ceh-30 for the CEMs is the defining that balance.
So that in a hermaphrodite CEM, basically what happens is egl-1 is sufficient to cause the CEMs to die.
But in a male CEM, that balance is somewhat different.
And now, even with the egl-1 activity there, that's not enough to cause the cells to die.
In other words, we think ceh-30 is setting the sensitivity to the programmed cell death pathway of the CEM neurons, okay.
So that's the basic model.
Now, it is possible that in addition, ceh-30 acts by regulating egl-1.
We don't have data to preclude that, so we could be doing something that's analogous to what I told you about before, we were not sure.
But this is the basic model.
Now, one theme of what I told you about both yesterday and today is conservation, evolutionary conservation.
So what about ceh-30?
Well, ceh-30 has two mammalian counterparts.
And Hillel has taken the murine genes called Barhl1 and Barhl2 and tested them in transgenic worms for ceh-30 function.
And the answer is both have some, not perfect, but have some ceh-30 function.
So as I said last night, these suggest that there is conserved function from worm to mammal for these proteins.
Now, interestingly, one of these two mirror genes has been knocked out.
It's been done by Mengqing Xiang at the Robert Wood Johnson Medical School in New Jersey.
And mice homozygous for deletion of the ceh-30 homolog, Bar-- Barhl1 are deaf.
And they show a progressive loss of hair cells that's why they're deaf.
Our hypothesis is that this hearing loss caused by the loss of these sensory neurons in the ear is because Barhl1 like ceh-30 is a cell-type specific anti-apoptotic regulator.
And when you lose its function, you cause cell death of these particular cells, okay.
That's the hypothesis.
And in fact, Mengqing Xiang and colleagues have gone on and obtained data consistent with, but I would say, not yet category improving that their hypothesis is the case.
Okay, so we would end to all of this thinking we knew where we were going with regulation egl-1.
That wasn't the case.
And cancer genes, we ended up with deafness.
So how do we think about this together?
And the answer is it may not be quite that different particularly with respect to what's going on in human disease.
Because what I've told you is that in mammals, if you knock out this homolog, you get deafness.
But think about what would happen if you misexpress this cell-type specific, normally, cell-type specific anti-apoptotic gene in some other cell type?
There may be one or more cell types in a mouse or a person that if you confer this cell type, normally, cell type protective activity would still be capable of functioning.
And if so, it would protect.
And if it protects, it could lead to cancer.
So even though the normal function is in the nervous system to protect sensory neurons, it still could be an oncogene.
We don't know.
And so, the message I want to leave you with is just simply that is a hypothesis.
Any gene that is involved and the cell-type specific regulation of programmed cell death could, if misexpressed or miscontrolled in some way, could infect lead to human disease.
That's quite distinct from the normal biological function of the gene.
Okay, so having said that, again, I turn back to the overall pathway of last-- yet last night, I focused on this core killing aspect to the pathway and I now talked to you a little more about ced-4 short and ced-4 long.
And now, I've told you a bit about what's upstream with that core killing pathway and how specific cells decide whether to live or whether to die.
Okay, and then to conclude, I just want to say thank you to those people who have been in the lab over the years working specifically on this project, the project of programmed cell death.
And I'll stop with that.
Thank you very much.
[ Applause ] >> There's some time for questions.
[Inaudible Remark] >> Sure. Can we get light on, please?
If anybody has a hand up, make a noise, [inaudible].
>> Tra-1 in those head specific neurons in the males is also regulating egl-1 in keeping pathways-- >> That's all right.
That's all right.
>> So the pathway is turned off for two reasons-- >> In?
>> -- in those cells.
>> Tra-1-- >> -- is regulating both CH-- >> No, no.
Tra-1, what we know is Tra-1 is regulating ceh-30.
>> Right.
>> Okay. And that is sufficient to have come from the regulation.
We do not know if ceh-30 or tra-1 regulate egl-1 in that pathway.
Our guess would be that tra-1 probably doesn't because of the sign of the interaction.
The-- you know, but that's what always a caveat, you know.
If it's a repressor, it's the wrong side.
But there isn't any evidence that it is regu-- there isn't any biological evidence than it is directly regulating egl-1 for that cell type.
>> So the simple idea of the tra-1 and the cis-sited egl are doing the same thing [inaudible] neurons as here is wrong.
[Inaudible] two things there but they are behaving the same way in those sites.
>> Tra-1 is acting to control these two different cell-type specific sexually dimorphic cell deaths via two different targets, and probably via two different parallel inputs to the cell death process.
That's-- well, we can say with some confidence.
But it is possible that it is acting most likely indirectly because we know everything that tra-1 does, it does through ceh-30, okay.
So for anything we can see, we say it's ceh-30 mediated, but we can't rule out the possibility that there's a little effect of ceh-30 through egl-1.
There isn't any detectable effect that you can see of the tra-1 that isn't through ceh-3.
[ Inaudible Remark ] So the level of message is a factor of 30 looking at one shot of time integrated over the entire animal.
We have no idea what the level of message is in a cell that is about to live or a cell that is about to die.
So we only have total transcript above this.
[ Inaudible Remark ] The model we have but the basis for it is not-existent, is that you actually had interacting proteins.
So the short and the long form, and that's an antagonist.
That's not the only thing that's possible.
We have no data whatsoever to say [inaudible].
In fact, you know, I would say it's still a hypothesis that the in vivo function involves the alternative forms.
We do have evidence that there is a protective function.
We have still not proved rigorously that that protective function is the long form.
That's the simplest hypothesis.
[ Inaudible Remark ] Well, that was the one slide that I showed, was that we get-- in that experiment, we don't see the long form.
But again, those experiments have been, I would say, enticing.
I consider them extremely [inaudible] at this point.
>> If you would do DNA damage in adult worms, do you get the same sort of apoptosis you see and mammals than in-- so the pathway give any hints about what's going on in the DNA.?
>> Right. So the question is how about using this as a model for DNA damage?
And Scott Cameron who I mentioned in the couple of experiments I described is a pediatric oncologist and he came to the lab just studying radiation increased apoptosis.
And after his first experiments obtained [inaudible].
You know, people sometimes say that after the nuclear holocaust, all that's going to be left in the phase of the earth is cockroaches.
So they got it wrong.
It's going to be C. elegans.
It's very radiation resistant.
Having said that, you can induce apoptotic test by high dose radiation particularly in the [inaudible], okay?
And Michael Hengartner who was a graduate student in our lab is now a professor and dean [inaudible] in Zurich has been analyzing that pathway.
And there's the beginning of the story that submerging there.
But it's not easy and it's not terribly sensitive.
And the cell deaths that are discussed really don't [inaudible] by radiation [inaudible] apoptosis.
Yup?
>> So all the stuff we discussed was in neuron, was that because of your findings or did something [inaudible]?
>> Neurons are really good because there are a lot of different neuron types.
And so, that makes it easy to study.
And in most of the programmed cell deaths that occur are in the nervous system.
So there are 131 cell deaths, and 105 of those affect neurons, okay?
So it is basically the biology makes it most tractable within the context [inaudible] system.
And that's what we've been-- that's what we really focused on.
We-- [inaudible] what we can do [inaudible], we haven't approached [inaudible].
>> You said you didn't know whether ceh-30 works downstream egl-1 or whether it's going to activating egl-1 transcription?
>> No, ceh-30-- ceh-30 definitely functions downstream of egl-1.
What I can't say is it might not also function through egl-1.
I can't be sure it doesn't, but it certainly acts downstream with egl-1.
>> So if you were to express egl-1 like on a different promoter, it doesn't overwrite [inaudible] action [inaudible]?
>> So if you try to overwrite with a topic expression, my guess would be you could come off with a level.
We haven't done that experiment in that way.
But my guess would be that you could overwrite it, because I think it's part of the [inaudible].
[ Inaudible Remark ] We don't know because the problem is we can't-- we can make-- we can make the appropriate double mutants and analyze via-- up to the point of ced-9, but for technical reasons, we can't ask that question.
And we haven't been able to get the cell-type specific analysis of, for example, levels of genes and proteins that tell us that at that level.
So we just haven't been able to answer that question.
So if you look at the [inaudible] diagram, we say that we know the ceh-30 acts downstream of ced-9 whether it feeds into ced-4 or ced-3 or downstream of ced-3, or all of the above.
We have no basis for saying it.
We have experiments in the books that are ways of trying to get to ceh-30 target.
And so, if anybody's interested, let me know.
[Inaudible] try to get [inaudible].
It can be done.
It sound like you'd already been there.
We don't know what the target is and it's conserved.
So we think that's a whole new conserved mechanism to work out from that.
Yup?
[ Inaudible Remark ] In C. elegans, that is with essentially all-- almost all of the rest of the lineage.
Everything is reproducible for animal to animal.
We're talking here about developmental cell death within animal that has a very small number of cells.
And it's completely reproducible, which is one of the reasons why it seems so tractable.
When you look at cell death in other organisms just as when we look at cell lineage in those organisms, you get more variation.
You can even look at a bigger [inaudible] and not all that much bigger and you get more variations in what goes on.
Well, in C. elegans is reproducible.
[Inaudible Remark] >> No. No.
No. We-- they seem to be stochastic cell sometimes make through.
And we know a little bit about other inputs to the [inaudible].
We know, for example, phagocytosis isn't simply swallowing a dead cell.
It actually promotes the killing a little bit.
We know that there are set of transcription factors including some old friends like RV, E2F, and DP that promote killing by regulating presumably, transcriptionally, something we know [inaudible].
So we have a variety of clues of other inputs to killing where we don't know.
We haven't pulled it together yet how they're working together and how many [inaudible] pathways these are.
Okay, this is all I've-- everybody, thank you.
[ Applause ] [ Inaudible Conversations ]
About the speaker
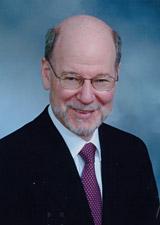
Robert H. Horvitz
David H. Koch Professor of Biology, MIT.
Nobel Prize in Physiology or Medicine, 2002.
Prof. Bob Horvitz is the David H. Koch Professor of Biology and a Howard Hughes Medical Institute Investigator in the Department of Biology and McGovern Institute for Brain Research at MIT. His pioneering studies have made him one of the central figures in research on programmed cell death ("apoptosis"}. In this work he discovered key genes that control cell death in C. elegans, a tiny transparent worm made up of fewer than 1,000 cells; which provides a simple, yet powerful model for cell biology and development.
Dr. Horvitz did his undergraduate work at MIT where he double majored in mathematics and economics, graduating in 1968. He then switched areas and went to Harvard to study biology. He first worked with Jim Watson, well known for his participation in discovering the double-helical structure of DNA and later with Wally Gilbert, an inventor of one of the first DNA sequencing methods. After receiving a Ph.D. in Biology in 1974, he went to the Medical Research Council Lab of Molecular Biology in Cambridge, England, to study neurobiology. There Horvitz teamed with others in Sydney Brenner's lab to trace the fate of each cell in C. elegans as it developed from an embryo into an adult., They found that cell division in the worm produced many more · cells than survived to make up the mature animal, suggesting that a systematic process might be killing the unneeded cells. In the mid-1980's as a biology professor at MIT, Horvitz identified the first "cell death" genes. As he has explained, "Discovering that programmed cell death is specified by particular genes established that programmed cell death is a basic biological process, much like cell division, cell migration, and cell differentiation." This work has already made an important impact in medicine as well as in basic science.
For his work on apoptosis and for his studies of organ development in C. elegans, Horvitz shared the 2002 Nobel Prize in Physiology of Medicine with Sydney Brenner and John Sulston. He has received many, many other awards including the Genetics Society of America Medal, the American Cancer Society Medal of Honor, the Charles Leopold Mayer Prize of the French Academy of Sciences, and recently, last year, the Mendel Medal of the UK Genetics Society and the Eli Lilly Lecturer Award.