Efraim Racker

Drug Development in the 21st Century or Are We Going to Cure All Diseases
Transcript
- >> Rick Cerione
-- individuals who were responsible for those achievements, and right from the very beginning, we've really been quite fortunate in terms of having had an outstanding group of lecturers, many of whom are Nobel Laureates starting with our first lecturer, Jim Watson, and then among others Sydney Brenner, David Baltimore, Harold Varmus, Paul Nurse, Mario Capecchi, and tonight's lecturer, Aaron Ciechanover.
We've had really an outstanding group and we've tried to identify those individuals whose contributions and scientific impact truly epitomize the breadth of Ef's research interest and the fundamental contributions that Ef's laboratory made, the basic biochemistry into a molecular understanding of human disease.
After an extraordinary career at the New York University and then at the Public Health Institute at New York, Ef was recruited here to Cornell in 1966 as the Albert Einstein Professor of Biochemistry, and he also served at the time as the Chair of what was then the section of biochemistry.
Ef's research interest were really be quite broad but in one way or another they all would seem to circle back to understanding the basic biochemistry underlying cell metabolism and then how alterations in metabolism can give rise to disease.
And he was especially interested in the connections between altered metabolism in cancer and particularly the phenomenon known as the Warburg effect which, of course, was discovered by another outstanding biochemist, Otto Warburg, referring to the altered glycolysis, this characteristic of cancer cells, and this is an area that's now giving a tremendous amount of renewed interest in the cancer biology field and that is something that Ef would be very, very pleased about because there was probably no better champion for the importance of metabolism in cancer than Ef.
And so what we hope is that the spirit of Ef's scientific approaches, his love for basic research, and really how fundamental discoveries can be applied to alleviate disease and human suffering will continue on through these lecture series, and we're so specially pleased to have Aaron tonight as this year's Efraim Racker lecturer, and I know Ef would be pleased as well because the way that Aaron really worked out the enzymatic cascade, its underlying protein ubiquitination fit perfectly with the style and the way that Ef did his science.
And so with that, David Shalloway will now introduce our lecturer.
- >> David Shalloway
Aaron Ciechanover was born in Haifa shortly before the establishment of the State of Israel, and took biology in an early age starting with the love of plants, turtles, and lizards, moving on by the age of 11 to experiments on osmosis observing the expansion and contraction of onion epithelium in saltwater solutions.
His interest in biology meshed with practical application in medicine and he received his MD from the Hebrew University Medical School in Jerusalem in 1972.
After three years of service, he's a doctor in the Israeli Military.
He made, as he says, "the most important decision in his career" to go into surgery or to enroll in a graduate school and start a career in scientific research.
Clearly, he made a good choice, and he began his graduate studies with Avram Hershko at the Technion in Haifa.
There over a period of five years and in collaboration with Irwin Rose at the Fox Chase Cancer Center, he began the discovery and unraveling of the system of ubiquitin-mediated protein degradation, work that he continued as a postdoc in MIT.
It was an interesting choice of topic, interest in the pathways leading from the synthesis of DNA to the synthesis of RNA to the synthesis of proteins and the mechanisms regulating these synthesis pathways was high.
But not much attention had been given to the other side of the coin, the degradative pathways that balance against the synthetic pathways in protein control.
Many probably thought that there was not much sophistication to be found on this side of the equation, but this view itself has been degraded over the years, and thanks to the work of Ciechanover and others, we now know that the system has enormous scope and have also complex network of thousands of components.
The study of this network has involved-- evolved into a subfield all on its own, and professor Ciechanover has been at the forefront of research in the area.
And for his discoveries, he's received numerous awards, you can read about them in your program, but they include the Lasker Award, the Israel Prize, and the Nobel Prize.
Apparitions of the Ubiquitin Proteolytic System are now known to be involved in many diseases.
And Professor Ciechanover's laboratory, the Cancer and Vascular Biology Research Center of the Technion, the Israel Institute of Technology is currently engaged in studies of the relationship between protein degradation and cancer.
We're delighted to have him as this year's Racker lecturer in Biology and Medicine [ Applause ]
- >> Aaron Chiechanover
Good evening, everybody.
It just happened so that I knew Ef Racker in person.
I was a graduate student, and he had a sister in Haifa, in our city.
As you all know, he was Jewish that escaped Austria just before the rise of the Nazis to power, and went to England and then from England to the United States, well-taken in the United States and then ended up landing in Ithaca, and he contributed enormously at that time to the discovery of the ATP synthase complex.
He discovered the two-- the first component of the ATP synthase, and then the mechanism which then made support to the theory of Mitchell about the gradient of hydrogen ions that drives energy and production by the mitochondria, production of ATP.
So he was really at the forefront of biochemistry and mostly how we are converting our nutrients into the common coin of energy which is ATP, as I said, he came several times to Haifa.
I don't know if knew me but I knew of him.
And as David mentioned, and he really was-- he constructed or reconstituted the system in vitro from different components.
And this is the way that biochemists are working to this very day and that's the only way to understand how complex mechanisms are there.
If you want to understand how a mechanical watch is working, the only way is to take it into parts, put all these parts in the clock and then put them together, and if that watch will work after you put them together, then you know how it works.
And, you know, molecular biology and all these wonderful techniques are all assisting tools but they are not any means by themselves because at the end of the day, we need to understand the basic mechanisms of complex pathway.
So I'm truly honored to give this year a memorial lecture of Efraim Racker.
So the ubiquitin system that you've heard, I'm going to talk tomorrow in a regular departmental seminar, and since this is the evening and it's an open lecture to the public and you are probably representing a broad audience coming from different disciplines, I thought to entertain you-- not to entertain you the funny way.
There was an idea that I like a lot and that's the evolvement of personalized medicine.
Everybody talks about it, it's a big buzz and many people-- they have their hope in it, but it's a very complex idea.
And I will just take you not to the technicalities of it takes, maybe we will touch on it but really, what does it take?
What brought us to this point and what's beyond the point?
How simple or complicated personalized or person-targeted medicine is and going to be.
So obviously, I mean, the big dream of everybody and of society maybe is to remain young forever and you see there are many movies with Mel Gibson, and the idea didn't find itself only to-- just to movies and to the entertainment world but even to books, and many people are working on it.
And actually, when you think about it, why not?
We are deepening our knowledge, why not?
We should, you know, like we eradicated infectious diseases, not really, but at least, we partially defeated them and some diseases completely, and disappeared-- polio, for example, disappeared from the healthy world and many other diseases.
Why not the cancer and/or the degenerative diseases and many other diseases will be defeated?
And we shall not need medical schools anymore, physicians, hospitals.
But with it, obviously, we should live life of high quality to a much longer lifespan, I mean, we should live maybe 200, 300 years.
And actually, if you think about it, lifespan is still expanding-- extending.
In the 20th century, we really took it in an exponential curve from about an average of 50 years at the beginning of the 20th century back to about 80 at the end of the 20th Century, which is amazing.
Because if you think about it in the old times, in the Greek times, the Roman, the Egyptian times, people lived about 25 years on the average to 30 years.
So it took 4,000 years from 2,000 before Christ, B.C., to almost 2,000 A.C. to extend life by only 25 to 20 years, and then in one single century, we took it 30 years further, which is amazing.
And we know why, we know that it's medicine mostly, x-ray, antibiotics, understanding of clean life, sewage system, and nutrition, what's healthy, and hospitals' development and so on.
So, now we are a little bit leveled off this exponential curve.
We are now adding one month of life per year, that's what the numbers show us.
So people think thought if the cure will continue to behave like that, the people that are being born these days will have extra six to seven years on what we are living, but then it goes on and on and on and on, and we don't know how the curve is going to deflect or to behave in the future.
So this is not something that is foreign to us or strange to us.
But the real answer is that we don't know.
But people are dreaming on it and this is coming from the Time Magazine that came out immediately after Judah Folkman, a famous pediatric surgeon from Harvard Medical School, came with the idea of angiogenesis.
And angiogenesis is obviously, you know, we are now relating to it as a general phenomenon as growth of blood vessel vasculature but he came to it with the idea of how to treat cancer, and the idea was, let's forget about cancer, why do we delve into the problems of cancer?
Let's cut away the oxygen lines to cancer, the blood supply, by understanding why tumors attract vessels to grow into them, and once we understand it, once we isolate the growth factor, the angiogenetic factors, VEGF and all.
What we know if we shall cut their line of supply, the vasculature will collapse, will degenerate, and we shall solve the problem regardless of the cancer.
Well, we are still living with cancer, and in a big way.
And we-- now that we know how to sequence genes and we can differentiate between malignant tissues and then the healthy tissue, we know that even the endothelial cells that grow into cancerous cells are cancerous by themselves.
So the epithelial cells, the vasculature is malignant by itself.
So the idea of stopping it actually is doomed to fail, while there is Avastin today, you know, there are drugs that can treat it but the beneficial effect are minor and marginal, and-- because you have a tumor that lives side by side with another tumor.
So we are in the problem, we are not outside the problem.
His idea was to get outside the problem but he didn't manage to, and obviously, the dream to have an X on cancer is far still far away.
But even if we will put an X on the cancer, remember there are no free lunches.
So life expansion from 50 to 80 costs us with the diseases because a hundred years ago, people didn't die from older generation.
Why? Because they didn't live long enough to have it people didn't die of cancer, why?
Because they didn't live long enough to have it.
True, there is cancer in children but I can tell you that cancer in children is about one to two percent of all cases, and the rest of the cases, 98 percent, are people that are 60, 70, and 80 or so.
It's disease, it's an age-related disease, pharynx and lungs.
If you draw the curve, you would see right directly.
And the same for all degeneration and the same for degeneration because myocardial infarction, the result of chronic developing, evolving atherosclerosis is a degenerative disease, and other vascular diseases are also degenerative diseases.
So basically by taking life from 50 to 80, we opened up almost half of the new textbooks of internal medicine and other professions of medicine.
So, even if theoretically, maybe not theoretically, we shall defeat cancer in older generation and we don't know what's behind the corner, I mean, we just-- we need to keep on working.
So, let's-- don't entertain ourselves with imaginary ideas and dreams and get it down to earth to treat the problem that are we are facing.
But, you know, if you ask medical students-- if you go to a class, I'm teaching myself classes of medical students, and you ask them what do you want to do when you grow up?
I mean, you want to be surgeons, yes, half of the class wants to be surgeons.
Cardiologist, another 70 percent, where we are already beyond a hundred percent [laughter], and neurosurgeons, brain surgeons, 80 percent, and they want to go into this fleshy, you know, sexy professions, heroic, when blood is splashing out of the patient [laughter] in the operating theater.
You ask them who wants to go to public health for epidemiology or aging or geriatrics, you will find a single hand raised, a single finger.
Why? These are the problems that you we are facing.
Geriatrics, the aging population, nobody, I mean, who wants to deal with old people, nobody.
[laughter] And I mean, it's sad, actually, it's sad, and public health.
Now, if you walk into a hospital these days into an average American Hospital, and American societies, typically, Israel is the same per percentage, you will see that by simple measures, half of the patients shouldn't have been there.
Half of the patients should not have been in the hospital.
They could have been avoided their diseases simply by behaving differently.
Think about this woman, this is suicidal woman, I mean.
People that feed themselves about 10,000 calories per day are aiming to die, that's what she wants to do, fine.
We cannot avoid it, but it's an avoidable, preventable disease.
And obesity is truly epidemic and you know what obesity leads to, people didn't think about it, people thought that obesity is only hypertension and heart attack, no, obesity is cancer.
There is a tight linkage, a strong linkage between obesity and cancer and we now also start to unravel the mechanism.
This disease, smoke, nobody forces anybody to smoke, and again, people think of lung cancer, but it's not only lung cancer.
It's lung diseases at large, it's degeneration of the alveoli and reducing immensely the surface of absorbing of air in the lungs, and there are many other diseases, brain diseases that are common with smoking.
Think about the environment.
If we shall live in a cleaner environment, think about East Europe where East Germany and Russia immediately after the fall of the Iron Curtain and the communist regime, awful industry didn't care at all about the environment, the rivers, the soil, nothing.
You know, we are contaminating our environment, and then infectious diseases.
So many-- if you look, if you go into the hospital, you'll see that half of the people that have heart attacks and obesity and diabetes and kidney diseases and many cancer that could have been avoided and so on and so forth.
But, you know, we are generating problems, we are expanding life, we are, you know, running sophisticated laboratories strength to dig in to sophisticated mechanisms.
And on the other hand, we are-- I'm not talking about road accidents and I'm not talking about shooting in wars and I don't know, like [inaudible] and drinking and whatever troubles that we are bringing in ourselves but-- which are also included in this number that could have been avoided.
But probably, we are human beings that it's all-- that all of these diseases can be grouped under one umbrella called behavioral diseases.
So, it's all to our behavior, but let's-- you know, let's still go deeper into drug discovery because I think the revolution of personalized medicine comes from a necessity, you know, we always have a necessity.
So we need to understand the rules.
And we'll talk less on the personalized medicine, sequencing genomes, mutations, protein-- or posttranslation of protein unfolded, I will not bother you with this.
I just want that we shall just understand what's the dynamics of the process.
So let's think about drugs and we are now in the third revolution of drug development but let's talk about the first revolution.
Think about it, biomedicine is the youngest of all professions in science.
Physics and chemistry are much older but biomedicine, as we know it now, enzymes, you know, think about DNA, the big deal, you know, all these genomes that you are talking about and sequencing and a whole human genome in one day for less than thousand dollars.
It was only 1944, 70 years from now, the DNA was discovered as the material that transmits genetic characteristics, genetic features.
It was the biggest discovery of the century probably by Avery at the Rockefeller Institute with McCarty and MacLeod-- and Maclyn McCarty and Macleod.
So, you know, it's a 70-year-old discovery that we know that DNA has to do with inheritance.
It was discovered in bacteria, actually, not even in eukaryotes.
And then the double helix took another 10 years for the double helix and then the big explosion.
But biomedicine, clinical experiments as we know it nowadays are barely 60, 70 years old.
So there was medicine before and there was [inaudible] fermentation and the Egyptians and the Greeks and the Chinese used medicines, but it was all kind of a barefoot type of a physician and medicine like herbs and so on.
But there was nothing like communicated medicine, that everybody know of, that aspirin is the drug to reduce fever or to combat inflammation.
And there were clinical trials 10,000 patients, 10,000 got placebo, well-controlled experiment, these are all new creatures of basically the last few decades.
We know about them until the '20s and the '30s and until then it was all kind of erratic type.
So the first revolution, so drug development is condensed basically to the last 78 years in a methodical way, and the first part of it was what I call the era of incidental discoveries.
Take for example the aspirin which is probably the most used drug in the pharma industry.
Most of the faces that I see here are young, but I'm sure that a few of you are taking the aspirin, me too, but for no good reason just because I have a physician friend and I'm a little bit concerned.
But the aspirin is probably the drug that is the most successful drug in the pharma certainly by tools of utilizations.
So, tons of aspirins are being used yearly by millions and millions of people worldwide.
And the aspirin was known to the Egyptians in willow bars, the bitter material of willow bars that you will be taking ache and taking pain away and then it further developed but it wasn't really until Felix Hoffman in the '20s who worked for Bayer, a German pharmaceutical company, had his father having rheumatoid arthritis which is a debilitating disease.
It's inflammation of the joints.
People are basically paralyzed, they cannot even button up, they cannot dry themselves after shower, they cannot walk.
I mean, it's a debilitating disease, and he remembered the old literature on pain-relieving material and he synthesized aspirin which is acetylsalicylic acid in the basement and purified it and gave it to his father.
Naughty son, going-- cooking, something in the basement and give it to his father as a drug, and lo and behold, not only the fever goes away and the pain goes away but the inflammation goes away.
Now, we know because it inhibits cyclooxygenase, the enzyme that's involved in biosynthesis of inflammatory mediator of-- different inflammatory mediator, prostaglandins and others.
And the aspirin came to the world then meanwhile, we never-- research on aspirin never ended up.
So it's the best anti-inflammatory that we have.
Well, there are steroids and many known steroid anti-inflammatory drug, NSAIDS, but aspirin is really a very good one.
It has side effects, gastrointestinal bleeding.
But now people have realized that if it is anti-inflammatory, maybe it's also an anti-cancerous drug because inflammation and cancer are really tightly knit.
And it's [inaudible] with chronic inflammation, think about Crohn's disease, chronic inflammation of the colorectum and so on.
Inflammation leads to cancer.
These two are a duo, twins, the amazing twins in medicine.
So people are now thinking of giving aspirin as a prophylaxis, as a preventive drug even for cancer.
But certainly for heart attack, it looks like Clinton but it's not Clinton.
[laughter] But certainly the guy here has a heart attack and people are now treating all patients with heart attack afterwards with aspirin in order to prevent the aggregation of platelets.
Platelets are the small cell that leads to blood coagulation.
Many people including myself are taking aspirin even before they had the first heart attack but that's a matter of choice, but aspirin is the routine, the standard, the gold standard treatment of patient following myocardial infarction.
And you can imagine that myocardial infarction or heart attack is a very, very, very common disease.
So we are talking inflammation, we are talking cancer, we are talking heart attack, and aspirin came by incidence, complete incidence, coincidence.
I mean, nobody researched it, nobody did any clinical trial, all kinds of literature accumulated, Felix Hoffman, his father wonderful story, here it is.
Another drug is penicillin.
Again, serendipity.
Sir Alexander Fleming, later on joined by Sir Ernst Chain, and Florey, but he was a microbiologist, and for those of you, I believe that many of you have grown bacteria on a Petri dish even for just transforming bacteria.
You know how bacteria look on the other, and he left the dish on the table open for the weekend and went home.
And it's true, you know, it's not a fable, this is still in the British Museum and the original-- this is not the original.
And when he came back after the weekend, he saw that the spore that was in the air just fell on the dish, grew into a fungus, and then he noted-- everybody else like me, I would have thrown it to the garden.
You know, you come in the morning, after you smeared the dishes, you look, contaminated, out, transformation didn't work, out.
[laughter] He noted a halo here in which bacteria don't grow and he surmised that the fungus secretes an antibiots, an antimicrobial material, antibiotic, against life.
And how-- that's how penicillin came to the world and then people said, "Wow, if one fungus secretes one antibiotics, maybe another one secretes another one," and then streptomycin came, Waksman, and so on and so forth in the whole world.
This was probably one of the major revolutions in medicine, introduction of antibiotics because people died of simple scratches in the battlefield, women died of simple birth just because of tears in the vagina.
So, I mean, the introduction, it allowed the expansion of surgery and then the impact of introduction of antibiotic to medicine is just unbelievable.
It's probably underestimated.
And, again, serendipity.
It doesn't say that it wouldn't have been discovered, otherwise-- but the root is serendipitous.
Let's go to the next one.
The next one is a revolution that we are still in and it's called high throughput, I call it non-intelligent brute force screening.
[laughter] Why are we screening?
We don't know what we are screening but-- I think that this is a reflection of the development of chemistry.
Remember '50s, '60s, and '70s, chemistry really evolved.
People-- chemists would synthesize whatever they want as long as it doesn't violate the laws of thermodynamics.
And many compounds were accumulated and people started to make libraries and so, and then people said, "Wow.
If they are libraries, let's try them on." Research can be cultured in small ways, robots go to the incubator, pull out the dish, put a little bit of every material on each drop.
Some artificially intelligent software can read the dishes and let's find leads, it's not a drug, it's a lead.
And then the chemist will come and multiply the lead and toxicity, this is not a drug, we still need another billion dollar to make it a drug, but the beginning is screening, and we are doing a lot of small molecules screening today.
You are doing it now.
You are going to-- now you can find universities not only in the pharma, many universities have small molecules screening facilities and so on and so forth.
We don't know the mechanism, we don't care, initially, then we go back to the mechanism.
But initially, we screen, million compounds, hundred thousand compounds and so on.
So-- and many drugs came out of it, very sophisticated drugs came out of it.
I'm not cynical about it at all.
I'm just looking at the mirror and ask myself, "What have I done?" I'll give you one example, another blockbuster.
This is not only [inaudible] blockbuster but it's dollar-wise blockbuster.
Last year, statins which inhibit cholesterol biosynthesis were sold for 25 million dollars.
Unbelievable, and the patent is over.
Just collectively all the companies that are making, that are making it sold it for 25 billion dollars, nine zeros after the 25.
So-- and statins came by screening.
Actually, Akira Endo, he won the Lasker Award for medical research few years ago, screened for anti-cholesterol producing drug for another purpose, he wanted to develop an antiparasitic compound.
He screened the library of natural compounds not synthetic compound, it doesn't matter, that there was the same input and assay.
The whole idea about screening is making an output assay.
You need to have a rapid assay and he found statins.
And then statins developed to become better and better and better and they are truly a blockbuster, and again, the impact of statins on economy, on morbidity and mortality is amazing, you know, how much heart attacks are prevented and so on and so forth, second one and even first one.
And, again, there was a recommendation by the American Heart Association even to take it prophylactically for certain people, but I will not go into all those indication.
By the way, for those of you who are not in medicine, heart attack is occlusion of the arteries that bring blood to the heart itself by accumulation, gradual accumulation of cholesterol.
It's called atheromatotic lesion or atheroma.
[Inaudible] it's a whole-- there is a whole pathology inflammation in the margins, I will not go into it but gradually, there is a clot developing in the coronary arteries themselves, so the blood supply to the heart itself is ceasing.
So the heart doesn't get blood supply and obviously, it cannot contract then people are collapsing.
Now, let's go to the third one, and here we are.
This-- the first one is medicine and it's called the revolution of the 4Ps.
The 4Ps-- I should give credit to Lee Hood.
That's his and he put it together and it's personalized but together with it, it's going to be predictive.
We should know what else is coming to these patients.
If it's going to be predictive, maybe it could be preventive.
If we know the patients are going to develop something, maybe it could be preventive also and it's going to be also participatory because patients will take part in any decision that is being made.
And why they will take part in these different issue, patients are now much more learned and there is the internet, they are questioning.
Medicine stopped to be the profession that I studied about 30 years ago patronizing profession.
I am the doctor, trust me.
No, nothing like that, and there are legal suits and law suits so there are many developments that led to the fate that medicine becomes a participatory discipline.
So 4Ps, it's called the revolution of the 4Ps and the 4Ps belong to Lee Hood.
So what raised the, you know, and it's-- basically, I am not going to tell you the real details because I don't want to go into it because this will be like you've heard it zillion times from zillion other people, but it's going to be based originally basically on our genomes.
And our genomes are different, we all know that, and they are mutated and they have either mutation that are blind, snips and whatever our mutations that are important, that are expressive mutations.
And it's going to be based on the DNA but the need for it, the need for it came from the feeling that medicine today is very primitive.
Its like, you know, you can have-- everybody can have his own metaphor.
For me-- and I brought you some metaphors, I'm just entertaining myself putting this lecture together.
This is a pajama.
What is a pajama?
Pajama is not a suit that you go.
Pajama, there are three sizes, small, medium, large, I assume.
And then the rest is adopted by the rubber band.
People can just play with it.
It's not going out to a fancy restaurant.
Medicine today is like one size fits all.
And they-- you can go-- oh, this is the movie, I will not show you the movie.
And they're the same, you know, I found these ones that, you know, there is not one size motorcycle for people or there is not one size shoe for all.
And it tells that our classification of diseases is very-- is wrong in many ways.
We say breast cancer.
Okay, so a woman has a lump, she come to the doctor and-- but then we realized and I showed you how did we start to realize that actually there is breast cancer A and B and C and D and E and we don't know definite number.
And we wrongly classify all these diseases under one umbrella.
Only now we start to unravel the fact that actually we are dealing with a group of diseases that the end expression is a tumor but then with the rest, they are very different.
And the same for prostate cancer and talking mostly of cancer, I don't know the variety but what I'm talking to you is-- will be mostly related to cancer.
But there are many other diseases.
Why certain people will develop a heart attack at the age of 30?
Some will do at 40 and some will not-- will never develop it and most will develop it at the age of 70.
Why certain forms of Alzheimer are genetically inherited and some are onset or sporadic onset?
What is it?
It just shows us that Alzheimer is a different entity's complex that just shows up externally as a disease of forgetfulness or whatever.
But if some are genetically-inherited or mutation-related and some are sporadic, then it needs to be different.
By definition, it needs to be different.
The problem with medicine is it relied a lot on phenomenology, on the phenotype of the disease rather than on the mechanism because we don't have-- we didn't have a hands-on on the mechanism.
And as strange as it will look to you, what is the most useful tools in medicine, any medical-- yeah, there are some medical professionals here?
Yeah, and is biopsy-- you know what is a biopsy?
Biopsy is a needle.
You put a needle in the tumor, lets say breast, you take a sample of it out, you put it in the microscope, you stain it with hematoxylin eosin and you magnify it by 200 and you say, "Yes, this patient has cancer A, B, and C." And this technique has been with us for almost 200 years.
Did it change a bit, a bit?
Microscopy-- like microscopy when there are 20 thousand genes in the human genome, 22 thousand different proteins plus splicing zillions of splicing product in tiny mutations and we are using the microscope to tell the characteristics of the disease.
If you think about it, you're shivering, you're really shivering.
What we-- but that's it.
I mean, I'm not blaming anybody.
It's not that-- it's not the shortage of knowledge or stupidity of people.
It's the shortage of this current state of art of medicine, we just don't have better tools.
So the end result is the following obviously.
We have a group of women that has breast cancer or a group of men that have prostate cancer or whatever, and they come to the clinic at a certain day and they start to move along the line.
They're treated whatever, lumpectomy, mastectomy, chemo, radio, and then five years into the picture, it depends on the cancer, obviously.
Each cancer is different.
Let's say that the green one are completely cured, they are disease-free and the yellow one and the blue one are dead.
But, you know, the way from here to die is a really convoluted way.
You are not-- it's not easy to die.
People are suffering with-- from metastatic diseases, the side effects of chemotherapy.
Basically, we didn't stay here.
We were colorblinded to make this distinction.
We started the all-in-one treatment.
And some-- for some, it worked and for some, it didn't work.
So we failed to diagnose subgroups of diseases.
That's what we did.
Let's assume that the disease for all of them were at the same stage, didn't metastasize, whatever, it doesn't matter, we shall not go to clinical criteria.
So something is wrong about our diagnosis and we realize it.
And then we thought, okay, maybe it's the DNA that will tell us the story.
Well, we are far away from it but that's the beginning.
It's a roadmap but the roadmap becomes more and more muddier as we move.
And then comes a variation in the drug response, it's called ADR or adverse drug reaction.
You know that close to 150 thousand Americans are dying every year-- every year, 150 thousand Americans, of adverse side effects in the hospital, let it be contamination, it doesn't matter and I'm umbrella-ing it.
I'm putting it under this.
We, you know, we ask patients before we treat them, let's say, with penicillin, are you sensitive to penicillin?
So, if they know it, they tell, "Oh yeah.
When I was a kid and I took some tablets, I had a rash." So these are out, we don't treat them with penicillin.
But patients are developing all the time drug react-- adverse drug reactions and we cannot predict it.
We just, you know, we try and then they have it.
And it's a major problem.
It's called iatrogenic medicine.
Iatrogenic medicine is that we are making the patient sicker than they came to us to ask for help.
It's a disease made by-- it's a physician-made disease but the physician is-- I'm not talking about negligence, I'm talking about naive approach to the patient.
And there are many, many determinants that caused these adverse drug reactions.
You know, it could be environmental, nutritional, or genetic, whatever.
So we want to understand it.
We don't want to be in the lottery.
We want to predict ahead of time on a mechanism based what will be the fate because for now, it's statistics.
If the patient with a breast cancer will ask the doctor, "Tell me doctor, what is the chances of me to survive?" He will tell her 60 percent, 70, 80, it doesn't matter, he will throw a number.
And then she'd ask him, "How do you know doctor?" He said, "What do you mean how do I know?
I'm 75 years old, I've been in this clinic for 50 years, I've seen a thousand patients like you, I recorded each and every one of them and this is the statistics." So she is into a lottery but then she ask him, "What about me doctor?" And he says, "I don't know.
We shall wait and see in five years and then you will be on this side of the statistics or the other." Well, it's an imaginary conversation, but not really imaginary because realistically, it is a conversation.
And this is all about, you know, all these drug effects are just the difference between some basis in the DNA that determine that somebody will be sensitive to a drug and somebody will not be sensitive to a drug.
So let's go back to breast cancer and see how-- for instance, medicine is not something that you are going to get up one morning and see it coming.
It's in the door already.
It's there.
But it's a gradual involvement.
There are many tools.
Eric Lander is running now and I saw that he gave one of those talks in the past years, this huge genome-- sequencing genomes, different diseases project.
It's an international consortium.
So it's going on in parallel by a different route and different path all over the place.
But look at this, so again, the same picture.
Now, we try to predict.
We take-- here, you have woman A and woman B. We used to chemically stain the biopsy from the breast.
Oh, it's a needle biopsy, we take out, put it on the microscope, but now, we don't stain it, we just-- we stain it with an antibody.
And this woman is stained for an estrogen receptor with a non-specific antibody and this woman doesn't have it.
So this woman can be treated with Tamoxifen which is an antiestrogen receptor.
It will be a waste to treat this woman with Tamoxifen, why?
She doesn't have a mutated estrogen receptor.
She doesn't have a driver to the malignant process.
So now, we start to stratify, you see?
So already, we have at hand group A and group B and we can reveal to the woman that have the EGF receptor, the HER2 receptor that is mutated in some women, it's not mutated in other women.
So for those that it's mutated, we can treat them with an antibody called Herceptin.
The antibody will cluster the truncated receptor on the cell surface and will push it into the endosomal and the-- vacuole lysosome vacuole-- sorry, mutagen into the lysosomal pathway.
So we push the receptor that signals nonstop from the surface even without there being [inaudible] engaged, we push it inside by an antibody against the cytosolic, against the ectoplasmic thing.
So, this is Herceptin.
So, again, we need-- we shall not treat with Herceptin.
I'm not talking about money.
Women that don't have truncated HER2.
So we can have women that are HER2 positive but estrogen-receptor negative.
Well, this is positive or this is a negative-- or positive for both and then there is the progesterone receptor.
So we already, you know, think of the combinations just with three, how it can stratify them in play.
And then unfortunately, I'm less interested in this group because we have partial solution for that.
I'm interested for the triple negative woman, those that don't have mutated estrogen receptor and don't have mutated progesterone receptor, and don't have a mutated HER2 receptor.
What do we do with those then?
And they, unfortunately, don't have a treatment.
They have a disease that is most-- more aggressive than any of the others.
So these are called the triple negative.
But maybe there are more than triple, maybe there are quadruple, quintuple and hextuple.
The progress to finding those markers were found by coincidence by good a researcher that studied different system.
Now, the idea is to find it systematically by screening, by sequencing, and by knowing really the whole repertoire.
That is not going to be simple and it's not going to be-- well, that's the adverse drug reaction.
I will stop here.
Let's go back.
And this is the human genome obviously and we go via the human genome, but obviously, you know, we are not here.
We are at the end here because of its protein that are mutated.
Mutations lead to mutated protein but at the end, the proteins that are-- so, you know, knowing what's going on here will help us only partially, but at the end, we need to know here, and, you know, but it's evolving because there is metabolomics and protonics.
All these fields are evolving rapidly.
Some are more difficult.
It turns out that genomics is the easiest one because DNA is a linear information for [inaudible] very little modifications, methylation.
The language of proteins is much more complicated and the technology is a little bit behind but it's coming.
The technology is coming.
And we shall know what's going on, and-- but it's difficult because-- and we can-- actually we can treat in each level.
We don't have to wait in the protein.
We could-- if we want to treat a mutation, potentially we can do gene replacement.
And for the transcriptome, we can do, you know, we can handle the-- either science indeed by sRNA or understanding micro RNAs.
And here, we can do enzyme and protein replacement.
Think, for example, [inaudible] disease.
We have taken-- we are treating patients with say, [inaudible] disease.
We are making the enzyme in the laboratory in large amount and we give it to-- we inject it to the patients.
Obviously, we cannot give it orally but there are also upcoming oral technologies and we are injecting it to the patients via a wonderful pathway and of the mannose receptor, [inaudible] receptor, these are going into the lysosome and do their job.
And the-- but they are going-- it's not going to be straightforward, even this is not going to be straightforward, why?
Because at each level we are encountering problems.
And, you know, with genetics, we have epigenetic control obviously.
I'm not talking about histones and nucleus but I'm talking about methylation and changes-- chemical changes in the DNA.
And here we have control of [inaudible] with proteins, it's endless.
It's just endless.
The post-translation or modification.
And from phosphorylation to acetylation to oxidation, methylation, glycosylation you name it and you'll find it.
And to develop techniques that will tell us dynamically what's going on with all these-- it's going to be cumbersome but it's calm.
So, actually it's not a straight map.
And this map of sequencing of just getting the genome, it's going to be much more complicated, but again, and this is the project of Eric Lander and so on.
I don't want to bother you with this.
I just want to end up and open up a discussion of-- beyond the scientific complications, there are many other obstacles on the way.
And let's just see them and the last one I really want to expand into.
So many diseases are multigenic.
Think about the big hoo-ha that is running these days on autism.
You know, the whole spectrum of autistic disorders.
Just if you read nature from yesterday, there is the whole section, just on autism on the verge between science and science fiction, and what people are saying and not saying.
But many diseases are multigenic and we're getting confused, you know, we are doing a genome-wide association studies that are not-- people are not sure where they are taking them.
It's all statistics about linkages, but diseases, you know, we are out of the era of monogenic diseases.
You know, these were nice, Gauche, Niemann-Pick, Tay-Sachs, some metabolic diseases, some hypercholesterolemias and we are into more, you know, the complex diseases, mostly, the psychological diseases, depression, and I am talking huge sectors of the population.
I mean, we are talking, you know, each of them is like, 10, 15 percent of the population in different degrees of severity.
So, multigenes, and what do we do with multigenes, and how do we know which is the, you know, the main driver and which is the hitchhiker and which is the second driver, and so on and so forth.
Then, malignancies are really problematic.
Those malignancies, you know, we have genomic instability, because, you know, there is the theory of clonality of malignancies, but then once the clone evolves, differences are developing the different mutations and we find indeed in the human genome when once we are sequencing new malignancies we find numerous mutations.
In melanoma, there are about 100-- more than a hundred mutations, true.
Many of them belong to big pathways, signaling mostly.
But some don't and we still don't know which role each play and the problem with cancer is that is also unstable.
So, people, you know, if you follow the discussion of where to go in cancer, you know, the genome of cancer versus basic research, you will find different opinions.
I mean, the genomic people all go there.
You ask Bob Weinberg, he says that it's a waste of money.
Bob was also-- he was once one of the lecturers.
And he said, "We have to go to the major switches.
We don't care about cancer and let's go to apoptosis, to invasion of apoptosis.
Let's go to signaling, let's go to major pathway.
Forget about dealing with one or two mutations 'cause it will never end, will never bring a cure to it." So, there is-- there are big discussions and especially now in the United States, when the NIH money is limited, and people are bothered where the money should go, and then, so these discussions are extremely valid discussions.
Now obviously, human experimentation is very complicated.
In the end, we are experimenting real humans.
It doesn't matter how many mice we cured of cancer and we cured millions of them, I can tell you.
[laughter] Mice are mice and human are human, and they have different-- not only in life span, diet, genetic variability, I mean, there's no comparison at all and we fail, from time to time we fail and we see.
Think about the hormonal replacement therapy.
So, I put it here with HRT.
You know, initially people said, "Oh, let's compute here the age of the woman stop their cycle at 45, 50, let's add some hormones and let them go, be nice and young for another 30 years." And then, people started to scram and then they started to develop breast cancer more, not all.
And then, but if you don't give it to them, then they develop earlier atherosclerosis, and then you tell the woman, "Choose, you know, you have a choice.
What do you want?
You want hormonal replacement therapy with breast cancer or myocardial infarction without breast cancer?" So, I mean, I'm joking, but-- and nobody can make the choice because it's 1 in 10,000 and so on and so forth.
And then people are rethinking and now-- what-- hormonal replacement therapy was almost the standard 10 years ago, now, no, it's the choice of the woman.
She consults, she thinks, the doctor offers her choices and so on and so forth.
The same for stents.
Stents are these supporters of the coronary arteries that has springs that you put into via an imaging via catheter under the scope.
You are putting it under the string.
You are putting it to the coronary artery and the spring just expands and open the lumen.
People said, "Wow, it's fantastic." We open the lumen.
It was until now included with atherosclerosis, but then they learned that it's a metal and it causes some wounds and the wounds are scarring, and the scar is really obliterating the artery.
So, then they say, "Let's-- let's say, this mark.
Let's put it, but let's impregnate the material, they impregnate the plastic gold-- let's impregnate the material with anti-proliferative material.
Let's call it the drug-eluting stent, a DES." And they put tropomyosin, they put also both of the proton-pump inhibitors.
They did all kinds of method.
And they said, "Wow, fantastic." And then initially, indeed the patients responded well but then after two years or three they develop heart attack.
It's a complication of the stent.
Why? Because it never-- it never scarred and the wound that happened there in the intima of the endothelium that was there in the clot just sloughed off and occluded the artery.
So now, the cardiologists are looking very carefully at the coronary angiogram.
And they decide which stent is fitting each patient.
This is personalized medicine in a way, it's fitted to the patient.
It doesn't have to do with DNA.
But now, we are cooling down, we felt that, you know, the big magic days are gone and we are really looking at each and every individual patient.
And then cost of developing new drugs is the-- and obviously, there are lack of faithful animal models, you know, again, mice is you know, how can you test the neurodegeneration of a mouse?
Parkinson, yeah, the mouse can learn a little bit in the maze, to swim right, to swim left but this is not really neurodegeneration.
So, we let really-- and then obviously, the cost and the law suits, I mean.
And Merck developed this wonderful drug, Vioxx or Celebrex, that was develop by Serono and this is an anti-inflammatory drugs, fantastic drug and then it turned out that it has side effects but very rare side effects.
Development of myocardial infarction and then the patient sued the company and it has cost the company hundreds of millions of dollars and their shares went down, plummeted down so the company took it out of the shelve.
It's a great drug but, you know, people don't, you know, it's not that I'm asking people to care about the rest of the community but, you know, we are looking at the society.
We are living in a society and then what happens?
In a society, some people sue and then the company takes it away and then millions of people don't enjoy it anymore.
So it's like-- and then the board fires the chairman because the shares went down and restart the whole chain reaction.
And then what's really frightening is that the end of the blockbuster drug era.
For now, the companies, the drug companies, they are in real trouble.
The drug companies don't, you know, they're making billions of dollar but on the other hand, will take [inaudible].
And-- but they are building on blockbusters, statins, you know, big blockbusters, Gleevec, you know.
They are very much concerned that the big diseases will be now sliced down.
There will be breast cancer A, B, C, D and E and F and they will have to develop new drugs for all these small modern disease, small groups.
It will cost them a lot of money and the big blockbuster era is going to triple, no.
So for now, we are still there, we don't know what to do but it's coming, it's certainly coming.
Think about the triple negative, the one positive, the two positive, the duo positive, the triple positive, just with the three markers that they give you for breast cancer, we are already in three drugs in different combinations and it's going to be much worse.
So we don't know, and people are thinking of and the drug companies have a problem by themselves because they will not go to the third world anyway.
Think about antibiotics.
The drug companies don't develop antibiotics.
They are not interested in it because they know that the bacteria will develop resistance, it will cost them half a billion dollar per drug and then it will last for two years and go away before they see the first payment.
Certainly, they don't care about malaria.
What do they care about Indonesia and then Vietnam and I don't know what.
Who will take them the money to develop drugs to malaria?
So I think that the whole pharma industry will lead at the end some reshuffling.
Maybe governments will have to take over, I don't know, I'm not trying to solve it.
This is all-- every subject that I touch is a whole subject of its own but the last one is really a problem.
Well, all of them are problem but this one, I want to highlight and this is the bioethical problem of availability of genetic information.
I mean we are used to give genetic-- we are used to give the information to our doctors and the doctor knows a lot about us, lots of intimate things that we don't know.
We don't want other people to know, we don't want our employer to know that we are sick and so on.
There are diseases that's unfortunately in my opinion, social stigma on them.
Psychiatric disease have social stigma.
Certainly, we don't want the community around us to know that, I don't know, Schizophrenic under treatment, depressive under treatment, I don't know, manic depressive, psychic, whatever.
Infertility in families, you know, we-- there are cert-- but the amount of information the doctor will have with the DNA available will be immensely larger than any information that they asked us when we walk into their office.
'Cause now, they ask us that this, that that, what are the problem, and so on.
It's limited in scope but it's going to be much, much larger once the DNA reveals or maybe not now because we cannot read all the DNA.
But we shall be able to read it once all the GWAS will be finished, genome associate or the whole genome association studies in multigenetic 10, 20, 30, 40, 50, I don't know how long.
We shall know to read the DNA much better than we can read it now.
And a lot of the information will be there about our future diseases and so on.
And the dangers are huge, I will not go into to the government, the insurance company will want to slice insurance policies, spouses would like to know on one another.
Even now, we want to know whom we marry, and then so if we want to marry somebody that has two eyes, we don't have to ask questions, we look at the eyes and we see that our partner has two eyes but maybe next time, we want to know how the sequence of the DNA looks like.
What's the difference between something that we see and something that somebody can test in the lab and tell us?
No difference in principle, why there should be?
There isn't.
But even this is-- well, society will have to adapt to it.
But they'll-- I want to bring you just the problem that is unsolvable and we really don't know and it's not frightening but it's just there and with that I'll end.
Think about a woman that had a breast cancer and the breast tissues are fine.
The, you know, the surgeon took lymph nodes from the armpit and there is no metastasis and everything is fine once you got the treatment.
And then the physicians called her back to the office and said, "You know what, there is a genetic test that I can do for you that if you have a mutation in a certain gene, you are susceptible to breast cancer and the gene is called BRCA-1." We know the gene, called Breast Cancer number 1.
Actually, it's a ubiquitin ligase, but.
So but not only-- there was a very interesting science paper last week showing that if you cut off the ubiquitin ligase, the ring finger domain which-- there-- the ubiquitin is still active as a tumor suppressant, so it's very interesting.
The two separate functions that we thought until recently [inaudible] but I don't want to talk about it.
Anyway, so the physician is-- the patient asks the doctor, "But I had a breast cancer, you took it away.
And then now, I don't have anymore of the breast." He said, "Yes, but you have a second breast." So, you know, it's not a joke at all.
And many people in the United State that are carrying-- many women, that are carrying the gene are doing preventive mastectomy.
Actually, 80 percent of the world is in the states.
In Israel, we are still far away from this number.
We are trying in the Israel Cancer Society to raise the awareness of that.
So let's say that the woman, you know, she is shocked with this but, okay, she agrees at the end because she understands that she is a ticking bomb.
And then she comes back to the office but the doctor said to her, "You know, on top of it, the gene carries the risk-- a high risk for a much more deadly cancer.
It's called ovarian cancer because it's going to get on this axis.
The breast and the ovaries are on the same axis.
Well, ovarian cancer is really a deadly cancer.
Usually, we are talking high mortality rates.
And I offer you to take out the ovaries." And then she thinks and her husband and everything and she agrees to do it.
She is 50 years old, she has children already, she is not planning another and the problem is not ending.
She has a doctor.
Here comes the problem.
And the daughter-- 50 years old woman, she had a daughter of 25, whatever.
What the daughter of 25 is doing?
She's going to college, undergrad, grad, whatever.
And she has a boyfriend and she may marry one day in the next few months.
Now what we are doing with the daughter?
Are we hiding from the daughter the fact that the mother is carrying a gene that bears high risk for ovarian and breast cancer?
By hiding it, we are risking her life.
Are we telling her that the mother has it which means that the daughter has to do the test herself?
And what does it mean for the daughter?
Let's say that she's a carrier of the gene as well.
Will she take the breast out?
Will she hurry up marrying and having children and then take the breast and the ovaries.
And what the boyfriend will say, what the bomb he is marrying?
Again, you know, the BRCA is there, that's it.
BRCA is there, I'm not telling you anything.
It's not science fiction.
The gene is there and in 10 minutes test in the lab, we know whether it is mutated or not.
The gene is there.
So, personalized medicine is there, the problems are there, we'll have to make new choices because new horizons are going to be opened.
Some of them will be difficult but we handle the-- many other difficult choices in our lives and we shall handle this as well.
So again, I didn't want to bother you with personal-- with the technology of personal medicine, I just want to highlight it from different directions.
And one last word about this lecture, about this Ef Racker memorial lecture.
You know, different people have different choices, philanthropists, they want to see buildings, and I think that in my opinion, and I like philanthropists that are giving to people, the young people, that support research.
But you need to be visionary to do it because what researchers are doing, they are creating papers.
People cannot see that they are also creating knowledge and interaction and they amplify the knowledge by students and it's going from generation to generation.
I think that this is really a wonderful way to remember Ef Racker by a live lecture on science and maybe better than having a building with a plot that nobody will ever remember who was the man.
It's a live thing, it's amplifying 'cause I'm talking to people and then they will probably get an impression of what's going on and memorize it [inaudible] university in a science.
And I do hope that if Ef is looking on us from above, he's happy with what we are doing in his memory.
Thank you very much.
[ Applause ]
About the speaker
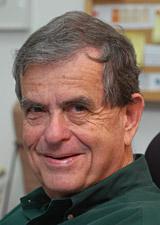
Aaron Ciechanover
Professor of Chemistry at the Tumor and Vascular Biology Research Center at the Technion-Israel Institute of Technology in Israel.
Nobel Prize in Chemistry (2004)
Born in Haifa, Israel in 1947. Dr. Ciechanover is a Distinguished Research Professor in the Technion – Israel Institute of Technology in Haifa. He received his M.Sc. in 1971, M.D. in 1973 from the Hebrew University in Jerusalem, completed his national service 1973-1976 as a military physician, and then continued his studies to obtain a doctorate in biological sciences in the Faculty of Medicine in the Technion 1982.
There, as a graduate student with Dr. Avram Hershko and in collaboration with Dr. Irwin A. Rose from the Fox Chase Cancer Center in Philadelphia, USA, they discovered that covalent attachment of ubiquitin to a target protein signals it for degradation. The group deciphered the mechanism of conjugation, described the general proteolytic functions of the system, and proposed a model according to which this modification serves as a recognition signal for a specific downstream protease. As a postdoctoral fellow with Dr. Harvey Lodish at the M.I.T., he continued his studies on the ubiquitin system and made additional important discoveries. It is now clear that ubiquitin-medicated proteolysis plays major roles in numerous cellular processes, and that aberrations in the system underlie the pathogenetic mechanisms of many diseases, among them certain malignancies and neurodegenerative disorders. Consequently, the system has become an important platform for drug development.
Among his numerous prizes, Ciechanover received are the 2000 Albert Lasker Award, the 2003 Israel Prize, and the 2004 Nobel Prize (Chemistry; shared with Drs. Hershko and Rose). He is a member of many academies including the Israeli National Academy of Sciences and Humanities, the American Academy of Arts and Sciences (Foreign Fellow), the American Philosophical Society, the Pontifical Academy of Sciences of the Vatican, the National Academy of Sciences of the USA (Foreign Associate), and the Institute of Medicine of the National Academies of the USA (Foreign Associate).