Efraim Racker

Cell Suicide: Programmed Cell Death in Development and Disease
Transcript
[ Inaudible Discussions ] >> Welcome all of you to the Efraim Racker Lectureship in Biology and Medicine.
And I'm especially pleased 'cause I am, every year, to be also welcoming Ef's daughter, Ann, and her husband John.
This lectureship was initiated in 1992 with the intention of bringing both to the scientific community and to the general public examples of the major developments in biology and medicine by those individuals who have been responsible for those contributions.
And in this regard, we've been really quite fortunate having been able to attract a truly outstanding group of lecturers, many of them Nobel Laureates, starting with our first lecturer Jim Watson and including Sydney Brenner, Harold Varmus, David Baltimore, Paul Nurse and tonight's lecturer, Robert Horvitz.
What we've strive for and I think what we've been able to succeed in doing is to identify those individuals.
His contributions in scientific impact truly epitomize with breadth of-- as research interest and the fundamental contributions that his laboratory made to basic biochemistry into a molecular understanding of disease.
After a distinguished career at New York University and then at the Public Health Institute of New York, Ef came to Ithaca in 1966 as the Albert Einstein Professor of Biochemistry and is the chair of what was then the section of biochemistry here at Cornell and has now evolved into the Department of Molecular Biology and Genetics.
It was truly an inspiration to a number of students and postdoctoral fellows who are fortunate enough to train with him.
Many of those would go on to establish their own outstanding independent careers.
I can tell you from firsthand experience, he was really a terrific mentor and a wonderful friend.
Ef did science as if it were an art for him.
He had this marvelous style of taking advantage of intuition and vision, every bit as much as technical details and literature knowledge, which probably should come as no surprise because another vast great love was art.
And really throughout his career, he would combine art and science in an almost seamless fashion and he was absolutely terrific in both of those areas.
That scientific interest were directed in understanding the fundamental steps of a variety of biochemical pathways, not the least to which being oxidative phosphorylation in the underpinnings of bioenergetics, but also in trying to understand how cell growth is normally controlled and how the lost of that control can lead to cancer.
And especially what he is interested in was this relationship between metabolism and the development of the tumorigenic state.
This is something that's getting greatly renewed interest now in the cancer community.
As many of you who knew Ef's kind of test, he have a presence that even some 17 years after he's passed, we really haven't been able to replace here at Cornell.
However, our hope is going at the sphere of that scientific approach, his commitment to basic search, and how fundamental discoveries can be applied to alleviate disease and inhuman suffering will live on through this lecture series.
And so I'm especially pleased tonight that we're hosting Dr. Horvitz who now be introduced by David Shalloway.
[ Applause ] >> It's a real pleasure to welcome Professor Bob Horvitz as the 2008 Racker Lecturer in Biology and Medicine.
You can read the basic facts about Bob's training and work in the program, so I'll just keep it short and add a few additional details.
Bob was an undergraduate in MIT where he double majored in math and economics, but the first and only course he took in biology was in his senior year.
Hope that doesn't discourage our early graduates in biology.
Yet this is not to convince him to change research directions in going to graduate school at Harvard in 1968 to study biology, even though he had never heard of the Krebs cycle at that time.
Quite a switch.
There he worked under the tutelage of Jim Watson and Wally Gilbert.
And moreover, it tells me, occupied the bench of the Honorable Paprov [assumed spelling] who was a co-graduate student and had around Professor Jeff Roberts as a postdoc working next door.
After graduating from Harvard, he went to the MRC labs in England as a postdoc to study neurobiology.
We've worked with Sydney Brenner's group on the development of a tiny worm C. elegans, where he collaborated with John Sulston, another postdoc.
Not only did Brenner and Sulston and Horvitz win the Noble Prize in 2002 for their work on C. elegans, but as previous mentors, Watson and Gilbert, were also prize winners-- Noble Prize winners.
It's clear that Bob knows how to choose good teachers, good problems, and good colleagues.
When Bob left England, his first visit as I understand at West of Cornell and to our credit, we offered him a job on the faculty.
To our dismay, he chose MIT where he's been ever since, at least we've got him back for tonight.
At MIT, Dr. Horvitz is the David H. Koch Professor of Biology and a Howard Hughes Medical Institute investigator in the Department of Biology, and also the McGovern Institute for Brain Research.
He speaks to us tonight on cell suicide program, cell death and development of disease.
Please join me in welcoming him.
[ Applause ] >> David, thank you.
More generally, I wanted to thank David Shalloway and also Flavier Vogue [assumed spelling] and Rick Cerione for inviting me to come and join you today and tomorrow.
It's a great honor to be this year's Efraim Racker Lecturer in Biology and Medicine.
I did have the privilege of meeting and talking with Ef Racker, but I never had the privilege of getting to know him as well as I know than any of the people in this room knew him.
I knew all about his landmark contributions to biology, strictly biochemistry, and as we heard, bioenergetics, which I learned about in the graduate school having not taking that direction earlier on.
It's also a great personal pleasure to be back here at Cornell.
I have long had many good friends here and I've gotten to see a number of people today.
I think I'll get to see a number of others tomorrow.
And it's always very special to have these personal of reunions.
I should note also that my visits to Cornell are often marked with interest just getting here.
The trip you were referring to, David, when I came and interviewed for a job, Jeff Roberts and I were reminiscing about that over dinner tonight.
And I was flying in from England and I went from England to JFK and then Allegheny Airlines had the, you know, I don't remember how many stops we did-- route between New York City and here, but what I remember was the last leg.
The weather was beyond dismal and the plane leaving New York City was like that, but we got to the first stop.
I think there was actually a second stop and then as they-- they got us off the plane, and as I got back on the plane, I heard the pilot say to the co-pilot, "I guess we'll give it a go." [ Laughter ] Now, you can ask Jeff what I looked like when I get off that plane that my preference would have been that they didn't do it.
Another visit here, I came here to give a lecture and I was coming from a meeting in Arizona, where that's actually the Muscular Dystrophy Association.
And Arizona was sunny, warm, swimming pool, bathing suits and it was February.
Then I came in at night and I think George Hess was my host, and I was suppose to come to the Statler Hotel, and the next morning, George was dismayed to discover I wasn't at the Statler Hotel.
Well, I got to-- he took a fine, I rented a car, went out on this blinding snow storm and ended up in a ditch.
[ Laughter ] OK. So, those were two previous visits.
Now, today, the journey here was much less eventful.
I flew into Syracuse, it was raining in Boston, no problem.
We got here and he had done a map quest inquiry to figure out how to come and I was-- took 81 south just like it said and I turned off on an appropriate road.
And I was going through a town called McLean, if I remember right.
And I'm going to straight on the road and it's a straight tube [phonetic] and there's a big sign up that says "Bridge Closed." [ Laughter ] And that's all the sign said.
[ Laughter ] So, next time I come, you'll have to give me some advice ahead of time as to exactly what the best way to arrive at.
Anyway, back to the topic at hand.
So I've been advised that this evening's audience is very broad.
So I'm here to try to present some very, very basic and actually mostly historical information about some of the work that we've been involved with over the years.
And I apologize to those of you who may find it a little bit simplistic.
I will be giving a research seminar tomorrow where you will actually see data and stuff like that.
But today, it's post-dinner, we're going to keep the lights on to like keep most of us awake if we can, and I'm just going to give you a bit of an overview.
Now, and as you heard, six years ago, I had the honor of sharing the Noble Prize in Physiology or Medicine.
And part for discovery is concerning programmed cell death.
Now, many people know that the Noble Prize comes with a gold medal and people also know it comes with some money.
But what people may not know is that it comes with a diploma, OK?
So this is my noble diploma.
And if you read it, and I assume you can read Swedish, if you can read it, what it says at the end is that this prize was in part for studies of the genetic regulation of programmed cell death.
And so, what I want to talk about for a little bit is what that actually means.
What do we mean by genetic regulation?
What is programmed cell death?
Why should anybody care that cells die?
And why is a prize in physiology or medicine awarded for findings in this area?
OK, so first genetic control, genetic regulation.
Genetics as I'm sure people in the audience know, generally, is the study of genes and genes are really important, OK?
They make us what we are.
They make us different from redwood trees and fish.
And they also make us different from each other.
And there are variations of genes that cause variations and particular attributes that we have, like eye color, blood type, and so on and so forth.
And other variations that can cause disorders, dwarfism or deafness or color or diseases that are like Huntington's disease is caused by a defect in a particular gene.
So, lots of things that genes do that are really important to us, and so we care about genes and we want to know how we can learn about genes.
And one way of course is to study our genes.
But we have limitations in studying the human genes.
For example, one way to study genes is to see how they're transmitted from generation to generation.
And what geneticists do in such studies is cross it, cross one individual with one set of genes with another individual with another set of genes.
And that's easy to do in a laboratory with people, we have to take what we get.
So there are limitations in that sense.
And there are other ways in which people are not, you know, the idea of experimental organisms.
So oftentimes, one studies genes in organisms that are much simpler and also very easy to handle in the laboratory.
Organisms like mice, fruit flies, single-celled yeast like-- are used to brew beer or make bread.
And another organism that's used in genetic studies is a microscopic round worm or nematode called Caenorhabditis elegans or C. elegans.
And we have many C. elegans experts here at Cornell, so I'm not going to go into details about C. elegans.
But it's a very beautiful little worm.
And I'll return to it in a few moments.
OK. So now, what do we mean by the genetics of programmed cell death.
And we can start essentially with what's a cell.
And in essence, a cell is the unit of life.
Our bodies are made of cells and they're made of many different kinds of cells, skin cells, nerve cells, muscle cells, and so on.
So what about programmed cell death?
Programmed cell death is synonymous with naturally occurring cell death.
It means cell death that in a sense is a part of the normal genetic program of an organism as it develops.
So what happens as an animal develops?
We start with a fertilized egg and it divides to make two cells and those divide over and over and over again generating a large number of cells.
For example, in us, something on the order of 10th to the 13th cells are made by this repeated cell divisions.
And then these cells take on specific characteristics to have particular traits and then they gather together to make tissues and organs like a nose or a heart or an arm or a brain, OK?
So those are the basic processes that occur during the development of an animal processes of cell division, cell differentiation, and morphogenesis.
In addition to these processes, there's another process that appears to be universal among the animals and the breathing wasn't recognized until much after the processes that I've been alluding to.
This is the process of cell death.
Quite remarkably, I think, many of these cells that are generated as animals develop do not survive, but they instead die.
And it's-- this death was natural death that it is referred to as programmed cell death.
And there are actually many examples of it and in many classic examples.
So for example, think about a tadpole.
Tadpole turns into a frog.
The tail of the tadpole regresses.
Those cells die.
That is programmed cell death.
Think about a human baby in utero and think about fingers and toes.
In utero, the fingers and toes each are connected to each other by webbing.
And that webbing is cellular and as the baby develops, that cellular webbing dies, programmed cell death.
For example, thinking about fingers and toes, think about birds.
OK, birds from this perspective come in two types.
Those that have webbed feet and those that do not.
Obviously, the web feet are good for swimming, good biological excuse.
What's the process?
The process is a differential regulation of programmed cell death.
In a duck, for example, the interdigital regions do not die.
In chicken, they do.
Birds with webbed feet, birds without.
For those of you who know Cambridge well, you've been recognized some of these birds.
The goose lives by the Charles River and the pigeon lives by some of the trunks where students get lunch.
[ Laughter ] I don't know either too personally.
Programmed cell death can be a very major event.
For example, in the development of a mammalian brain, as many as 85 percent of the nerve cells that are generated die.
In our immune systems, as many as 95 percent of the thymocytes, specialized blood cells in our immune system, 95 percent that are generated die, and die by programmed cell death.
Despite this widespread occurrence, for many years, programmed cell death was really overlooked.
And I think part of the reason was that biologists simply couldn't think about cell death as being something that an organism wanted to do.
So the thought was if cells were dying, something was going wrong.
They were damaged.
They were-- They couldn't be kept alive.
And this mentality came maybe from first principles, maybe from our personal experiences in the laboratory where we struggle to keep cells alive in order to study them.
If they died, we did something wrong.
So if cells die, something was wrong.
And it was in further discovery that this view was wrong that really led to the Nobel Prize, because what was found was that there is a biology of cell death.
Every bit is much as there is a biology of other fundamental biological processes.
Programmed cell death is an active process on the part of cells that die.
And there are specific genes that function to make cells die or to keep them from dying.
OK. Now, I've been saying a few words about the biology of programmed cell death as a natural part of biology.
But if you think about any part of human biology, if that biology goes wrong, it can lead to disease.
And programmed cell death is no exception to that.
Abnormalities in the control of programmed cell death have been associated with a wide variety of human disorders.
And this is, I'm sure the experts in the audience know, a short list.
There are many, many more disorders and they come in two flavors.
Disorders in which there is too much cell death and disorders in which there is too little.
For example, neurodegenerative diseases, Alzheimer's, Parkinson's, Huntington's, Lou Gehrig's disease, ALS and so on and so forth, all involve specific classes of nerve cells dying.
In some neurodegenerative diseases, the evidence is clear that these are in essence ectopic programmed cell deaths.
In others, there's probably partial growth for programmed cell deaths going awry.
In others, they may not be involved at all and still an open study.
But there's certainly are some cases of neurodegenerative disease where, basically, the wrong cells or the cells at the wrong time and place are expressing the program for programmed cell death.
On stroke, traumatic brain injury, which in this country means falling off from a motorcycle onto your head, aspects of age, myocardial infarction, congestive heart failure, and we can go on, all involved cell death.
And in many of these indications, the evidence is-- what's basically going on is that endogenous program that causes cells to die during normal development has been unleashed causing cells to die at the wrong time or in the wrong place.
The other side of the coin, these disorders in which there's too little cell death and examples of these include autoimmune disease where cells that should die don't and then go on to be able to be self-destructive to our bodies, and also cancer.
And cancer is an interesting example not only because it's so very important clinically.
People often think about cancer as too much cell division.
And that's certainly a correct way to think about cancer.
But it's only partially correct way.
If you think about the number of cells in our bodies, in the tissue, that number actually is defined by two antagonistic processes.
The process of cell addition by cell division and the process of cell depletion by programmed cell death.
If either process is perturbed, too much cell division or too little cell death, cell number will go up.
And in fact, there are certain cancers like follicular lymphoma, B-cell lymphoma, which clearly are disorders that fundamentally begin with the misregulation of programmed cell death, in that case, allowing B-cells in the immune system that should die instead to survive and that then allows a subsequent proliferation that leads to the cancerous state.
More generally, it looks like most and probably all cancers involve too little cell death, that downregulation and cell death amplifies the growth-- lost of growth control that occurs in cancers.
And furthermore, many of the traditional therapies that are used to treat cancers, radiation, chemotherapy, what they actually do is they activate an endogenous program of cell death and they kill tumor cells because tumor cells are ever so slightly more susceptible to these triggers of the endogenous cell death program.
One of the problems with the side effects from radiation treatment and chemotherapy is that others cells can activate of the cell death program as well.
OK. So having said all that, and the fact that programmed cell death is clearly important in biology and also clearly important in medicine, what was it that we did?
What did we find that led to the discoveries in this area?
And the key player in all of these, again, very familiar on this campus is this little one millimeter long microscopic, looks like about the size of a piece of lint nematode called C. elegans.
The two humans co-honored with me were Sydney Brenner and John Sulston.
Sydney introduced C.
elegans to biology and worked out the basic methods for the study.
He was interested in this animal because it was remarkably simple.
Remember, I said that humans have 10th to the 12th, 10th to the 13th cells?
C. elegans has 959, OK?
That's a big difference.
You cannot only count them, you can describe them in detail, every cell is known.
C. elegans is also very good for genetics.
I said that people aren't good 'cause you can't cross them, you can cross worms.
But more than that, they're still tiny.
You can get very large numbers of them.
You can grow a million worms with no difficulty.
And these worms grow up very quickly.
Geneticists aren't concerned with generations, you know, I've mentioned redwood trees before.
Well, it's a bad PhD project crossing redwood trees, right?
C. elegans generation time is three and a half days.
One week, you've got two generations.
So very, very good for genetic studies.
And Sydney had the inspired idea.
You could take this very simple, very genetic retractable animal and use it to explore basic problems in developmental biology and in neurobiology.
[ Pause ] John Sulston was a staff member of Sydney Brenner's lab, and John focused particularly on the developmental biology of the animal.
And knowing that it had very few cells, he started in the beginning with the fertilized egg and studied-- I'm now going to use my Ef Racker pointer, and studied the pattern of cell divisions starting with that single fertilized cell which divides to make two, each of which divides to make two again.
This is the Y-axis.
And this cell lineage diagram depicts the developmental origin of every single cell in the animals.
C. elegans is the only organism for which an entire cell lineage is known and this knowledge has provided a phenomenal background through the analysis of many problem-- many problems in developmental biology.
Compared what this slide would look like for human with 10th to the 13th instead of 959, and you can just get an idea of the kind of information that you can get and how powerful it might be.
Now, for those of you who haven't have the privilege of knowing John Sulston, this was a picture of him.
Right here, that's John.
And unfortunately, in this article, he's called by another name.
He is referred to as the Reverend Gabriel Burdett.
And according to the article if you read it, the Reverend Burdett is a proponent of a new theory, OK?
This is a theory that is an alternative to the theory of gravity, OK?
And specifically, the Reverend Burdett has quoted a saying, "Things fall"-- this is the intelligent falling theory by the way in case you missed the [inaudible] of it.
"Things fall not because they are acted upon by some gravitational force theory, but rather because a higher intelligence is pushing them down." OK. So, that's John.
And now, what's he doing in this particular article?
This article was published by "The Onion".
[ Laughter ] And it is a commentary on, you know, what.
The photograph is legitimate.
The real photograph and the real accompanying article is this one.
This was the day John Sulston announced the sequence of the human genome.
OK, so serious science was going on.
"The Onion" took advantage of John's books, I think, to label him in some other way.
OK, so back to the science and the cell lineage.
So John primarily was responsible for working out this cell lineage.
And what he discovered was that as this animal goes through a series of cell divisions that generate its 959 cells, it actually makes more cells than that, 131 more.
And they're made, but they're not found in the adult.
And the reason they're not found in the adult is that these cells die.
They undergo programmed cell death.
Now, the fact that programmed cell death occurs in this animal in a completely reproducible way, we know ahead of time what cells are going to live and what cells are going to die, made it possible to use genetics and Sydney Brenner's inspiration about how to use genetics to analyze the genetic control of programmed cell death.
And that's basically what my life did.
Now, if you look at the animal, the living animal, you can see cells that are in the process of dying.
Here is a dying neuron in the-- on the bottom side of C. elegans.
Very near is neural friends and relatives, but very distinctive, has a very obvious morphology.
And if you watch this cell in time, it appears in this stage of death and it regresses, and in fact, the cell goes away, it is gone.
[ Pause ] When one looks ultrastructurally with an electron microscope, there are again very characteristic features of these dying cells.
Most obvious here is the electron density, the darkness of that dying cell.
But when you look in more detail, it turns out that this programmed cell death in C. elegans look very much like the cell deaths that were described in just about the same time that John was first observing this in a process that was named-- and I should say for the audience with the word that has three syllables and starts with A and is pronounced in at least seven different ways, OK?
I will try to stick to one, two, or maybe three of these pronunciations and say, apoptosis.
But if you prefer a long day or a non-silent P or the original Greek which sounds very different indeed, that's fine too.
What it refers to is a particular set of morphological changes that occur as cells die, described first by John Kerr and from Wyllie and Alistair Currie in human tumor samples.
These are the cell deaths that normally occur in most tumors and that in fact if they don't occur, you're going to even promote tumor growth further.
So the fact that C. elegans programmed cell death look like this mammalian apoptosis, suggested to us that if we could figure out the mechanisms of program cell death using C. elegans, we might learn something that would be generalizable beyond this microscopic worm.
So that's exactly what we tried to do.
Look for genes that were involved.
I'll skip that.
And the first mutant that was actually identified was identified by John Sulston.
And he found a mutant in which there were little tiny nuclei from cells that were present where cells that should have died would have been had they not die.
It turned out that these were cells that underwent programmed cell death, but failed to degrade the DNA.
In the dying cells of the DNA, which is the way these cells are stained with the DNA stain, the DNA remained and this gave a finger print of dying cells.
The gene turned out to control a protein that is an enzyme called the nuclease because it chews up DNA and called the gene NUC-1, which perhaps wasn't the best choice for other reasons.
And that was the first gene that was identified to perturb the process of cell death.
But if you think about it, this step is really a mopping up.
It's getting rid of the DNA.
Essentially, one cells were dying.
We were interested in going earlier in the pathway.
Well, the next step earlier was discovered by Ed Hitchcock, who was first at the MRC, Medical Research Council Laboratory of Molecular Biology in Cambridge, England where I worked with Sydney Brenner and John Sulston.
And he identified two genes that were involved in programmed cell death.
And they're not required for degrading DNA in dying cells, but rather they're required for the engulfment of cells by neighboring cells.
OK, so if you think about an animal where it's got all these cells dying, what happens the corpses?
And the answer is, other cells come in and swallow them and take advantage of their dying neighbors, use they're nutrients, and get rid of essentially the debris that is there.
So this process of engulfment or phagocytosis removes dying cells from the body of the animal.
And what had Hitchcock discovered was two genes, which are involved in this process.
And those genes he named CED-1 for Cell Death Abnormal, CED, gene number 1 and CED-2.
And in a CED-1 or CED-2 mutant, what happens now is all of those cells that undergo programmed cell death and remember what's happening, they go through a very transient period in which the cell death morphology is visible and then disappear.
Now, the phagocytosis is blocked and these deaths remain and you can see them.
And you can see them.
What's really important here is using Nomarski optics.
You can see them in living animals.
So you can take an animal like this and immediately look at it and say, "Aha, here are all the cells that are undergoing programmed cell death," OK?
So again, this is a step in programmed cell death, the step of engulfment, but this wasn't the step that we really wanted to focus on either.
We wanted to know how it was controlled, how's-- how was it decided?
What actually happens to make cells die?
And a graduate student in the lab, Hilary Ellis, Hilary is the larger of the two here.
[ Laughter ] She thought about the following approach.
In a CED-1 mutant, you can see all of those cells that it started to die during the course of the life of the animal.
So she said, "Let's look for a mutant of the mutant in which the pattern in cell deaths is different, because we can now seal the cell deaths easily.
You just look and there it is looking at you like polka dots on a tie." So she mutagenize CED-1, looking for mutants that were abnormal in patterns of cell death.
And one thing that she found was a mutant in a gene called CED-3.
And what happened in CED-3 was that you could see none of these cell deaths.
And when Hilary analyzed the CED-3 mutant, she found two things.
First of all, the reason you couldn't see any cell deaths in this now CED-1, CED-3 double mutant was because the process of cell death never initiated it.
There were no cell deaths.
Secondly, from her genetic studies, she could conclude that the mutation in this gene CED-3, that prevented cell death, turned off the CED-3 gene.
It didn't activate CED-3.
So the conclusion from that was very simple.
CED-3 is a killer.
You need the activity of CED-3 to get programmed cell death.
And if you turn off CED-3, essentially, all of those cells that should die instead live.
OK, this finding was the finding I alluded to earlier, because this finding said that since programmed cell death requires the function of a specific gene, programmed cell death is an active biological process.
It's not simply cells being mistreated.
It takes a gene to cause cells to die.
Now, with the discovery of this gene, we proposed a genetic pathway for programmed cell death saying that the last step is the degradation of the DNA, step before that was that of engulfment, getting rid of the dying cell, and then CED-3 drives the cell death process, OK?
Many people said, "Great, you've done it.
There's the genetic pathway for programmed cell death.
Should biology ever be so easy?" It was not.
Meanwhile, another graduate student in the lab, Carol Trent was studying a process that we thought was completely independent from cell death.
She was studying more on behavior, and in particular, the behavior of egg-laying.
And she isolated and characterized a set of 145 different mutants that don't lay eggs right.
Now, you may wonder how we know that, different ways to know that, this didn't.
And one of the mutants-- and she was working with technician Nancy Tsung on this.
One of the mutants in the gene that we called EGL-1, egg-laying defective gene, number 1, turned out to be defective in egg-laying because the neurons that innervate the egg laying muscles don't work and the reason they don't work is they're not there.
And the reason they're not there is that they're generated and then they undergo programmed cell death, OK?
So, in one of these EGL-1 mutants, these neurons were missing and the animals bloat up, full of eggs.
So, Hilary discovered that if you make a double mutant between EGL-1, which doesn't lay eggs because the egg-laying neurons undergo programmed cell death, and CED-3, which doesn't do programmed cell death, then the neurons aren't going to die.
They survive and the animals lay eggs, OK?
So, just to say it again, in a wild type animal, it has these neurons called HSNs and it's not egg-laying defective.
Something happened there.
I don't know what.
In an EGL-1 mutant, there are no HSNs in the animal's egg-laying defective.
And in a double mutant, in which these neurons don't die because they can't die 'cause they don't have CED-3, the neurons survive and now the animal is not egg-laying defective, OK?
So that confirmed that the problem with EGL-1 was a problem with programmed cell death.
But more importantly, it gave graduate student, Chand Desai, an opportunity to screen for mutants defective and cell death in a different way.
Because what he did was mutagenize EGL-1 and now, look for animals that could lay eggs.
We know the CED-3 mutants that can't cause programmed cell death will allow the animal to lay eggs.
So he said, "Are there any other genes that are like these," OK?
And in fact, it's even easier than that.
You don't have to look for an animal that can lay eggs.
You can look for eggs 'cause any egg that's been laid has been laid by a parent who can lay eggs.
So, mutagenize EGL-1, look for eggs, and he got-- there's Chand.
He got new mutants.
He got more CED-3s and he got another gene called CED-4.
So our pathway now has two genes because CED-4 had all the properties of CED-3.
Like CED-3, it's a killer.
Then Junying Yuan, who was a graduate student in the lab asked the question, "Where do these killer genes, CED-3 or CED-4, act?
Do they act to control some kind of hormone that sends a signal and says, 'OK, die?' Or do they act within the cells that are going to die?" And what she found is that both of these genes act within cells that are going to die.
These genes are suicide genes.
And this finding said, "The program cell death, at least to this extent, is fundamentally a process of cellular suicide." Then Junying cloned the CED-4 gene to look at it molecularly and didn't look like anything for some years.
But five years later, in biochemical studies done in Texas-- in Dallas, Texas, in the laboratory of Xiaodong Wang, this was saying it didn't look like anything then.
Study the process of programmed cell death in the biochemical system, Xiaodong Wang and his coworkers identified a human protein that promoted programmed cell death like phenomena that had a sequence like CED-4, OK?
So CED-4 looks like this human protein, Apaf-1.
Junying and graduate student, Shai Shaham, also cloned and characterized CED-3, the first of our genes.
And it too was noble.
It didn't look like anything in the databases.
And we had already published the paper on CED-4 that said, "CED-4, the first killer gene doesn't look like anything," OK?
And so, we figured the popularity of an article that said, "CED-3, the second killer gene doesn't look like anything either," wasn't going to be all that great.
So we didn't publish it.
We waited.
And Shai checked the databases.
This was the time when database entries were coming fast and furious.
And it took about two years.
But then, something emerged and there was a hit.
And the hit, you can see, 10th to the minus 14th, pretty good hit, was a human protein called interleukin-1 beta convertase, OK?
This protein, ICE, IL-1 converting enzyme, was studied by two different pharmaceutical companies interested in human inflammatory disease.
It converts the cytokine in our immune system, IL-1 beta, into an active molecule.
And CED-3 and ICE turned out to be the founding members of a family of proteins, today, known as caspases, proteases that are responsible for cleaving other proteins.
And finding that CED-3 looked like a caspase suggested first of all the CED-3-- well, the CED-3 looked like a protease, suggested that CED-3 likely was promoting programmed cell death by acting as a protease, and we'll later show that indeed as the case.
And secondly, that since there is a human protein that is similar, that humans probably have proteins of this family that act to promote programmed cell death as well.
We now know there's a family of close to a dozen caspases in us and many of them had been shown to act to promote programmed cell death or apoptosis, OK?
So, this gave a mechanism.
This was the first biochemical mechanism for programmed cell death and that was shown in that way.
OK, so then, what do we have?
That I already told you and that is Ron Ellis.
Ron, another graduate student in the lab was looking for more genes involved in programmed cell death.
And he focused on a particular cell that normally dies and he looked for mutants that would perturbed the death of that cell.
And he found two kinds of mutants.
He found mutants that did perturb only the death of that cell, and that led us into a whole extensive study of how in particular cells decide I'm going to live or I'm going to kill myself.
And that's the science I'm going to really focus on tomorrow.
He also found many alleles, copies of CED-3, many of CED-4, and another gene which we called CED-9.
And he and a graduate student, Michael Hengartner, analyzed this gene, CED-9.
And it turned out that CED-9 placed a very fundamental role in the cell death process.
But unlike CED-3 and CED-4, it's not a killer.
It's a protector.
When CED-3-- When CED-9 is on, cells move, when CED-9 is off, cells die by programmed cell death.
And normally, what happens is particular cells are controlled whether or not CED-9 is on or CED-9 is off.
CED-9 is an anti-death gene.
It's a protector.
And Michael Hengartner cloned CED-9 to study it molecularly.
And I was at a meeting and he sent me a fax which says, "Guess who comes out on top?" And the answer was a human gene, a human cancer gene, a proto-oncogene known as BCL-2.
This is the gene I've already told you about that is responsible for human follicular lymphoma.
It causes cancer by preventing B-cells from undergoing programmed cell death.
It looks like CED-9, which prevents cells from undergoing programmed cell death.
And Michael Hengartner put human BCL-2 into a worm and showed the human gene protects against programmed cell death, and he put into a worm deficient in CED-9 and showed that the human gene could substitute for CED-9 in the worm.
Now, you hear about genes all the time, but no gene is an island.
Genes work together.
And the fact that the human gene could work in the worm, protect against cell death and substitute for CED-9, said it was interfacing with the same pathway in which it normally works.
These pathways must be shared, OK?
So, conclusion, CED-9 and BCL-2 must act in similar molecular genetic pathways.
OK, so now, what is the pathway?
So Shai Shaham and Michal Hengartner did a series of genetic and molecular genetic experiments and ordered these three genes I told you about.
And it turns out, CED-3, the caspase, the protease, the killer, there's the fundamental driver of cell death.
And this CED-4, another killer--
About the speaker
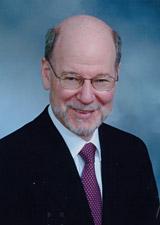
Robert H. Horvitz
David H. Koch Professor of Biology, MIT.
Nobel Prize in Physiology or Medicine, 2002.
Prof. Bob Horvitz is the David H. Koch Professor of Biology and a Howard Hughes Medical Institute Investigator in the Department of Biology and McGovern Institute for Brain Research at MIT. His pioneering studies have made him one of the central figures in research on programmed cell death ("apoptosis"}. In this work he discovered key genes that control cell death in C. elegans, a tiny transparent worm made up of fewer than 1,000 cells; which provides a simple, yet powerful model for cell biology and development.
Dr. Horvitz did his undergraduate work at MIT where he double majored in mathematics and economics, graduating in 1968. He then switched areas and went to Harvard to study biology. He first worked with Jim Watson, well known for his participation in discovering the double-helical structure of DNA and later with Wally Gilbert, an inventor of one of the first DNA sequencing methods. After receiving a Ph.D. in Biology in 1974, he went to the Medical Research Council Lab of Molecular Biology in Cambridge, England, to study neurobiology. There Horvitz teamed with others in Sydney Brenner's lab to trace the fate of each cell in C. elegans as it developed from an embryo into an adult., They found that cell division in the worm produced many more · cells than survived to make up the mature animal, suggesting that a systematic process might be killing the unneeded cells. In the mid-1980's as a biology professor at MIT, Horvitz identified the first "cell death" genes. As he has explained, "Discovering that programmed cell death is specified by particular genes established that programmed cell death is a basic biological process, much like cell division, cell migration, and cell differentiation." This work has already made an important impact in medicine as well as in basic science.
For his work on apoptosis and for his studies of organ development in C. elegans, Horvitz shared the 2002 Nobel Prize in Physiology of Medicine with Sydney Brenner and John Sulston. He has received many, many other awards including the Genetics Society of America Medal, the American Cancer Society Medal of Honor, the Charles Leopold Mayer Prize of the French Academy of Sciences, and recently, last year, the Mendel Medal of the UK Genetics Society and the Eli Lilly Lecturer Award.